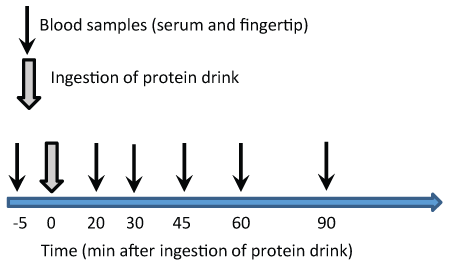
Figure 1: Timeline showing the timing of blood samples after ingestion of protein drinks. The same protocol was repeated for both protein drinks on separate days
Truls Raastad1* Markus Vagle1 Bomi Framroze2
1Norwegian School of Sport Sciences, Oslo, Norway*Corresponding author: Truls Raastad, Norwegian School of Sport Sciences, Oslo, Norway, Tel: +47 23262328; E-mail: truls.raastad@nih.no
Increased plasma levels of amino acids after ingestion of protein stimulate important biological systems, and especially changes in leucine concentrations has been linked to stimulation of muscle protein synthesis. ProGoTM, a salmon protein hydrolysate, is a protein that has shown good absorption in ex-vivo systems. The aim of this study was to compare the leucinemia observed after ingestion of ProGoTM against equal amounts of whey protein concentrate (WPC-80). The study had a randomized double-blinded cross-over design. Both products were given as 16 g protein doses to six young and healthy males. The first blood sample was collected just before ingestion of the protein, and thereafter at 20, 30, 45, 60 and 90 min. Blood samples were analyzed for glucose, insulin and two amino acids (leucine and glycine). The rise in leucine and glycine blood concentration generally mimicked the amino acid profile of the two proteins. A 15 min faster time to peak blood concentration of leucine was observed after ingestion of ProGoTM (28 min; 224 µmol/L) as compared to WPC-80 (40min; 339 µmol/L), whereas no difference in time to peak was observed for glycine (ProGoTM 38 min; 517 µmol/L; WPC-80 38 min; 284 µmol/L). The rapid increase in blood concentrations of amino acids after ingestion of both products resulted in an insulin response which reached similar peak concentrations (30 min; 124 µmol/L). To which extent the faster, but smaller, leucinemia observed after ingestion of ProGoTM has the ability to stimulate muscle protein synthesis remains to be investigated.
Aminoacidemia; Leucinemia; Glycine; Insulin; Absorption
Aminoacidemia is the increase in plasma levels of amino acids after ingestion of a protein-containing meal. The acute increase in concentration of essential amino acids in blood and tissue stimulates several important biological systems. Consequently, the acute aminoacidemia is regarded as an early indicator of specific protein-source efficacy, with respect to its effects on health and bodily function. The level of aminoacidemia is determined by the dose of protein ingested, the amino acid composition and the digestibility and absorption rate of the amino acids and short peptides present in the protein-source [1].
Several studies have shown that the rate of absorption of amino acids into the blood is modulated by the degree of hydrolysis of the ingested protein [2], the overall ash content [3], and type of protein source [4]. The salmon protein hydrolysate tested in this study has a moderate degree of hydrolysis at 28% and low ash content due to the method of manufacture, which uses mild enzymatic hydrolysis conditions rather than the typical higher temperature conditions used in other processes [5].
Both the speed and amount of amino acids absorbed post-exercise can have a direct beneficial effect on muscle protein synthesis rates [6,7], as well as on whole body post-prandial metabolic responses [8]. For the stimulation of muscle protein synthesis, changes in leucine concentrations after protein ingestion has been focused [7,9,10]. The relation between observed changes in blood leucine concentrations and the concomitant increase in muscle protein synthesis after protein ingestion, has led to the “leucine-trigger concept”; meaning that a faster and higher rise in blood leucine concentrations, and subsequent in intramuscular leucine concentration, triggers a greater increase in muscle protein synthesis rates [11]. Consequently, proteinsources that are rapidly digested and contain large amounts of leucine, like whey, has been suggested as potent stimulators of muscle protein synthesis [12]. Given that the”leucinetrigger concept” is correct, other protein sources giving a fast and large rise in leucine concentrations after ingestion should in theory, have the same anabolic potential in muscle.
The Norwegian salmon aquaculture industry has focused on using salmon offcuts after filleting to produce human grade nutritional products. At one such facility, the head and backbone offcuts are subjected to enzymatic hydrolysis followed by separation of the water-soluble protein phase and spray-drying the concentrate to produce a palatable human grade salmon protein hydrolysate powder (SPH). This hydrolysate contains oligopeptides and peptides with a maximum molecular weight less than 3000 Daltons, and gel permeation chromatography analysis has shown that ~50% of these peptides have a molecular weight of less than 1000 Daltons [13]. Consequently, the salmon protein hydrolysate ProGoTM has the properties to be rapidly digested and absorbed; resulting in a rapid aminoacidemia as initially shown in the in-vitro Tim-1 gastrointestinal model study [14]. However, the leucine content in ProGoTM is lower than in whey, and it is unclear whether the salmon protein can match the leucinemia observed after whey protein ingestion [15]. The aim of the present study was therefore to compare the leucinemia after ingestion of a dose of ProGoTM against the leucinemia after ingestion of equal amounts of whey protein in healthy young men.
The study had a randomized double-blinded cross-over design. All subjects met in the lab on two different test days and ingested one of the two protein drinks at each visit in a randomized order. There was a washout period of a minimum of 48 hours between each trial. The protein drinks investigated were ProGoTM and WPC-80. Both products were given as 16 g protein doses dissolved in water (100 ml). In adition, the subjects drank a glass of water (~150 ml) and chewed one sugar-free gum (Extra White; Wrigley Company) right after consuming the protein drinks. Written informed consent was obtained from all participants before the start of the study. All procedures and methods used in this study has been evaluated by and approved by the Regional Committee for Medical and Health Research Ethics in Norway (REC South East).
The protein proportions in the powders were 95% and 77% for ProGoTM and WPC-80 respectively. Consequently, a total dose of 16.8 and 20.8 g were used from the respective powders to get 16 g protein in each drink. The amino acid composition in the products is given in Table 1.
Per serving (16 g protein) * | ||
Amino acid content (g) | ProGoTM | WPC-80 |
Alanine | 1.2 | 0.9 |
Arginine | 1.0 | 0.5 |
Aspartic-acid | 1.3 | 1.9 |
Cysteine | 0.0 | 0.4 |
Glutamic acid | # | # |
Glutamine | 2.1# | 3.1 |
Glycine | 2.2 | 0.3 |
Histidine | 0.3 | 0.3 |
Iso-Leucine | 0.4 | 1.0 |
Leucine | 0.8 | 1.9 |
Valine | 0.5 | 1.0 |
Lysine | 1.0 | 1.6 |
Methionine | 0.4 | 0.3 |
Phenylalanine | 0.5 | 0.6 |
Proline | 1.1 | 1.0 |
Serine | 0.7 | 1.0 |
Threonine | 0.6 | 1.3 |
Tryptophan | 0.1 | 0.2 |
Tyrosine | 0.3 | 0.6 |
Table 1: Amino acid composition in the two protein products tested in this study
*Amino acid composition was not measured in this study and the given
numbers are therefore based on available information based on previous
analyses of the two products [14,15]. #, glutamic acid and glutamine in total
A total of six young and healthy males completed the study (Table 2). They were all physically active and free from any conditions affecting the digestive and absorption system. At each test day, the subjects met in the lab at 08:00 am after an overnight fast. After 5-10 min relaxation in the lab, the first blood sample was collected from an antecubital vein, in addition to a fingertip blood sample. Thereafter the protein drink was ingested. The entire drink had to be finished within 5 min (3-5 min). Thereafter blood samples were collected at 20, 30, 45, 60 and 90 min in order to follow the blood response for glucose, insulin and amino acids.
Age (years) | Body mass (kg) | Height (m) | BMI (kg/m2) |
25 ± 1 | 81 ± 6 | 182 ± 4 | 24.7 ± 1.4 |
Table 2: Subject characteristics given as mean ± standard deviation (n=6)
Blood samples were drawn into serum Vacutainer tubes. After clotting in room temperature for 30-40 min, the tubes were centrifuged at 3400 rpm for 10 min at 4°C. Thereafter, the serum was transferred into new tubes and frozen for later analysis (amino acids -80°C; insulin-20°C). In addition, glucose concentrations were immediately analyzed from fingertip blood samples (Glucose 201+; HemoCue® , Brea, CA, USA). Mean of duplicate values varying ≤ 0.2 mmol/L was used in the data analysis.
Amino acids (leucine and glycine) in serum were analyzed at VITASAnalytical Services. An aliquot of 20 µL plasma was diluted with water and propanol and a mix of stable isotope labelled amino acids added and used as internal standards. Samples were derivatized using propyl chloroformate and extracted into isooctane before analysis by GC-MS. Instrumental analysis was performed on an Agilent 6890 GC system with a split/splitless injector and a 5973N mass selective detector (Agilent Technologies, Palo Alto, CA, USA). Separation of amino acids were performed on a Zebron ZB-AAA analytical column using a Phenomenex EZ: fast amino acid mix for calibration (Phenomenex, Torrance, CA, USA). Insulin in serum was analyzed with Roche E 170 module at the Hormone Lab, Oslo University Hospital.
The change in blood concentrations from baseline to the remaining blood samples for each protein drink was analyzed with one-way repeated measures ANOVA with Dunnett’s post hoc test. Two-way repeated measures ANOVA with Bonferroni post hoc test was used to evaluate differences in blood concentrations between protein drinks. Furthermore, two-tailed, paired Student t-tests was used to test for differences between protein drinks in peak concentrations, time to peak concentrations, and differences in the area under the curve (AUC) Figure 1.
Figure 1: Timeline showing the timing of blood samples after ingestion of protein drinks. The same protocol was repeated for both protein drinks on separate days
Ingestion of WPC-80 resulted in a rise in blood glucose after 20 min, compared with baseline (p<0.05; Figure 2, left), whereas no statistically significant changes in glucose concentration was observed after ingestion of ProGoTM. The glucose response was, however, not significantly different between the protein drinks. Both protein drinks resulted in an insulin response peaking 30 min after ingestion (Figure 2, right). The insulin response and peak insulin concentration was similar after ingestion of the two protein drinks.
Figure 2: The glucose response (A) and insulin response (B) after ingestion of 16 g protein from ProGoTM and whey protein concentrate (WPC-80). *Significantly different from baseline (p<0.05)
The increase in serum concentration of the essential, branched-chained amino acid leucine, was larger for WPC-80 compared to ProGoTM (p<0.05), whereas the increase in blood concentration of the non-essential amino acid glycine was larger after ingestion of ProGoTM (Figure 3, p<0.05).
Figure 3: The leucine (A) and glycine response (B) in absolute values and values relative to baseline (C and D) after ingestion of 16 g protein from ProGoTM and whey protein concentrate (WPC-80). *Significantly different from baseline (p<0.05); # significantly different from the other protein drink (p<0.05)
The peak serum concentration of leucine was larger after ingestion of WPC-80 compared to Pro-GoTM (339 ± 35 vs. 224 ± 18 µmol/L, resepectively, p<0.05), whereas the peak concentration of glycine was larger after ingestion of ProGoTM (517 ± 85 vs. 284 ± 29 µmol/L, p<0.05, Figure 4). The different content of amino acids in the protein drinks was largely determinative for the observed differences in blood concentrations.
Figure 4: Peak leucine (A) and glycine concentrations (B) in absolute values and in values in percent of baseline (C and D) after ingestion of 16 g protein from ProGoTM and whey protein concentrate (WPC-80). *Significantly difference between protein drinks (p<0.05)
The mean time to reach peak serum concentrations of leucine was significantly faster for ProGoTM compared to WPC-80 (28 ± 4 vs. 40 ± 8 min, respectively, p<0.05). However, for glycine the time to peak concentration was similar between protein drinks (38 ± 8 vs. 38 ± 11 min, respectively; p=0.88; Figure 5).
Figure 5: Time to reach peak serum concentration of leucine (A) and glycine (B) after ingestion of 16 g protein from ProGoTM and whey protein concentrate(WPC-80). * Significantly difference between protein drinks (p<0.05)
The area under the curve (AUC) for serum concentrations of amino acids was calculated for the first 90 min after ingestion. For leucine the AUC was larger after intake of WPC-80 compared to ProGoTM (p<0.05), whereas ingestion of ProGoTM resulted in a larger AUC for glycine (p<0.05, Figure 6).
Figure 6: Area under the curve for serum concentrations of leucine (A) and glycine (B) for the first 90 min after ingestion of 16 g protein from ProGoTM and whey protein concentrate (WPC-80). * Significantly difference between protein drinks (p<0.05)
The main finding in this study was that the changes in blood concentrations of the investigated amino acids mainly reflected the amino acid content in the protein source; leucine increased more after WPC-80, whereas glycine increased more after ProGoTM. Compared to WPC-80, an approximately 12 minute faster time to peak blood concentration of leucine was observed after ingestion of ProGoTM, whereas a similar time to peak concentration was observed for the non-essential amino acid glycine. This indicates a possible additional mode of action for BCAA uptake from hydrolyzed peptides versus whole protein powders, but the lower leucine content in ProGoTM may also have contributed to the shorter time to reach peak blood concentration. Comparing serum levels of leucine 20 minutes after ingestion, ProGoTM showed a significantly higher serum concentration than would be expected from simply extrapolating from the content of leucine in both proteins. The leucine content in the ProGoTM drink was 42% of that in WPC-80, whereas the rise in blood concentration of leucine 20 min after ingestion of ProGoTM was 60% of the increase observed after WPC-80 ingestion (increase of 67 vs.112 µmol/l for ProGoTM and WPC-80, respectively). This supports a relatively faster absorption of leucine from ProGoTM compared with WPC-80. Interestingly, the non-BCAA glycine, did not show an increased absorption ratio at 20 mins or at peak levels. The rise in blood leucine to peak concentrations was, however, better reflected by the leucine content in the two drinks (increase of 85 vs 193 µmol/l for ProGoTM and WPC-80, respectively, 44% of the increase observed after WPC-80). Although leucine blood concentrations reached much higher values after ingestion of the leucine-rich WPC-80 product, limitations in the absorption capacity might have influenced the observed changes in blood concentrations. The high content of leucine in the WPC-80 product might have challenged the maximal absorption capacity in the specific transport system for branched-chain amino acids across the brush border membrane in the small intestine [16], whereas this was probably not the case after ingestion of ProGoTM. The rapid increase in blood concentrations of amino acids observed after ingestion of both products resulted in a significant insulin response which reached similar peak concentrations and affected the blood glucose responses similarly. The insulin response after protein ingestion may contribute to the anabolic response in muscle and other tissues [17,18], but increased insulin availability does not seem to have marked effects on postprandial muscle protein synthesis [19].
According to the “leucine trigger concept” it might be hypothesized that ProGoTM is a less potent protein source for the stimulation of muscle protein synthesis compared to whey, because of the lower peak blood concentration of leucine after ingestion [11]. However, it is not clear whether it is the rise in leucine concentration or the peak concentration after a meal, which is the most important factor stimulating muscle protein synthesis. Furthermore, we did not measure changes in intramuscular leucine concentrations in this study, and the intracellular changes are probably the direct stimulators of the initiation of protein synthesis. Several studies have shown that other amino acids are more important for the prolonged stimulation of muscle protein synthesis after a meal [20-22]. Consequently, the effect of the investigated salmon protein hydrolysate on anabolic responses in muscle cannot be estimated from the measured changes in blood concentrations of amino acids. Nevertheless, the shown specificity to rapid absorption of leucine indicates that ProGoTM is an interesting product for further investigations on muscular responses. It is therefore necessary to target further research towards studying the efficacy of ProGoTM for stimulating muscle protein synthesis after exercise, as well as study changes in the essential relevant biomarkers that might improve training adaptations and muscle recovery in individuals.
In conclusion, ingestion of ProGoTM resulted in a rapid aminoacidemia with peak leucine blood concentrations reached at faster times compared to WPC-80. Whether the faster time to peak leucine concentration with ProGoTM was a result of a preference to accelerate branched chain amino acid absorption over other amino acids, or simply was a result of saturation of the leucine absorption capacity after ingestion of WPC-80, could not be determined in this study.
The study was designed by Raastad, T, Vagle, M, collected most of the data, and data interpretation and manuscript preparation were undertaken by Raastad, Vagle and Framroze. All authors have approved the final version of this paper. Partial funding for this study was provided by Hofseth Biocare AS.
B. Framroze is working for Hofseth Biocare AS, the producer of ProGoTM
Download Provisional PDF Here
Article Type: Research Article
Citation: Raastad T, Vagle M, Framroze B (2017) A Randomized, Double-Blinded Cross-Over Study Comparing Increase in Blood Leucine Levels after Ingestion of Hydrolyzed Salmon and Whey Proteins in Healthy Young Men. Nutr Food Technol Open Access 3(2): doi http://dx.doi.org/10.16966/2470-6086.142
Copyright: © 2017 Raastad T, et al. This is an open-access article distributed under the terms of the Creative Commons Attribution License, which permits unrestricted use, distribution, and reproduction in any medium, provided the original author and source are credited.
Publication history:
All Sci Forschen Journals are Open Access