Figure 1: Schematic of search process for reviewing neurobiology of exercise in adults.
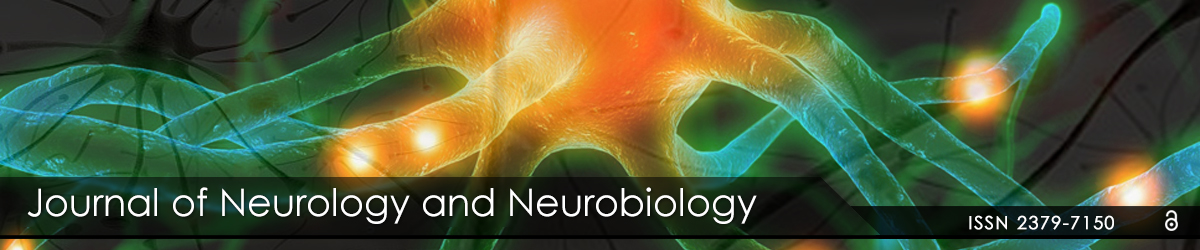
Full Text
David S Im*
Clinical Assistant Professor, Department of Psychiatry, University of Michigan Medical School, United States*Corresponding author: David S Im, Medical Director, Adult Psychiatry Inpatient Program, Michigan Medicine, Clinical Assistant Professor, Department of Psychiatry, University of Michigan Medical School, United States, E-mail: dvsm@med.umich.edu
Objective: Autism Spectrum Disorder (ASD) is a neurodevelopmental disorder characterized by markedly impaired social interaction and restricted/ repetitive patterns of behavior, interests, and activities. Maladaptive behaviors associated with ASD can include stereotyped, self-injurious, and aggressive behaviors. Current evidence-based interventions for ASD involve specialist availability, financial, time, and side effect constraints. Physical exercise has received increasing attention in the treatment of children with ASD, but research in adults is comparatively scarce. The purpose of this paper is to explore possible mechanisms by which exercise can reduce repetitive, self-injurious, and aggressive behaviors in adults with ASD.
Methods: The literature on the neurobiology of exercise is reviewed and compared with the literature on the neurobiology of ASD. Information from this comparison is then used to formulate possible mechanisms by which exercise may reduce maladaptive behaviors in adults with ASD.
Results: Plausible mechanisms for an exercise-induced beneficial effect on maladaptive behaviors in adults with ASD include modulation of neurotransmitter release and function, facilitation of endocannabinoid release, increased expression of neurotrophic factors, improved functional connectivity in higher-level cognitive networks, reduced oxidative stress, improved HPA axis function, treatment of comorbid depression, and reduction in amyloid beta deposition in cortical GABAergic neurons and subcortical structures.
Conclusions: Future research should attempt to confirm the efficacy of exercise in reducing maladaptive behaviors in adults with ASD as well as the proposed mechanisms for such a relationship. Verification of these issues would have major implications in terms of supporting an evidence-based, inexpensive, widely available, and broadly beneficial treatment for adults with ASD.
Autism spectrum disorder; Exercise; Neurobiology; Repetitive; Stereotyped; Self-injurious; Aggressive; Adult
Autism Spectrum Disorder (ASD) is a neurodevelopmental disorder characterized by markedly impaired social interaction and restricted/repetitive patterns of behavior, interests, and activities, with onset early in life, common co-occurrence of cognitive and communicative difficulties, and resultant negative impact on social, academic, and/or occupational functioning [1]. Maladaptive behaviors that can be associated with ASD include stereotyped motor behaviors (e.g. hand wringing or flapping), self-injurious behavior (e.g., head banging or finger biting), and aggression (e.g., hitting or kicking others) [2,3]. The complexity of ASD and consequent negative impact on functioning and quality of life, both for individuals with ASD and their families, warrants proactive efforts to identify effective treatments for its core symptoms and associated difficulties. While progress in this regard has been made over the last three decades, such interventions have primarily been studied for use in children with ASD [4-6]. Relatively little research has been conducted on effective interventions for adults with ASD [7,8]. While emphasis on early identification and intervention has resulted in larger numbers of children with ASD receiving services at an earlier age and improved trajectories in terms of language, social, and adaptive functioning [9,10], such emphasis has not addressed the needs of adults with ASD who may not have had the benefit of such early interventions. For these individuals, there is an equally compelling need for effective interventions to address the disorder’s core symptoms and associated difficulties in order to minimize morbidity and adverse outcomes (e.g., self-injury, violence).
One problem with current evidence-based interventions for ASD is that most of them (e.g., Applied Behavioral Analysis or ABA) require either the direct presence or regular involvement of specialists trained in administration of the specific intervention [11,12]. This may be difficult in settings (e.g., forensic facilities) or geographic areas where availability of such staff is limited. Even when such personnel are available, there are financial and time constraints in securing such interventions for individuals with ASD [13]. Other current evidencebased interventions for ASD have potential side effects; for example, antipsychotic medications for repetitive or irritable/aggressive behavior in ASD can cause sedation, weight gain, and metabolic syndrome [14].
Physical exercise is an intervention that has received increased attention over the last three decades in the treatment of individuals with ASD. Although a number of studies in children have documented beneficial effects of exercise on social functioning, [15,16] attention/ cognition, [17] and stereotypic behavior, [18-21] the literature on exercise for adults with ASD is comparatively scarce. A recent systematic review noted beneficial effects of exercise on physical fitness, motor skills, and general levels of stress and anxiety in adults with ASD, but did not examine the impact of exercise on core or associated ASD symptoms (including repetitive, self-injurious, or aggressive behaviors) [22]. The few studies that have examined the effect of exercise on repetitive, self-injurious, or aggressive behaviors in adults with ASD have shown encouraging results, albeit with small sample sizes [23-25].
While these findings are promising, further research is needed to confirm or refute the efficacy of exercise in treating problem behaviors in adults with ASD. In the meantime, this paper will consider a question that, to the author’s knowledge, has not been addressed in the literature to date: What are the possible mechanisms by which exercise could reduce repetitive, self-injurious, and aggressive behavior in adults with ASD?
The literature regarding the neurobiology of exercise in adults was reviewed and compared with the literature on the neurobiology of ASD (including in regard to repetitive behavior, self-injury, and aggression). Information from this comparison was then used to formulate possible mechanisms by which exercise may reduce repetitive, self-injurious, and aggressive behaviors in adults with ASD. Studies providing possible support for these hypothesized mechanisms are also cited.
Figure 1 presents a schematic of the search process used to review the literature on the neurobiology of exercise in adults. A search of the databases PubMed and Ovid MEDLINE from 2019 to 2024 was performed on March 11, 2024, using the search terms, “exercise,” “physical activity,” “neurobiology,” and “adult.” The search was limited to the last 5 years due to the inordinate number of citations (over 900) identified when using broader (e.g., 1987 to 2024) date ranges. Inclusion criteria included needing to have physical exercise as the main focus, needing to describe neurobiological considerations in relation to exercise, focusing on adults, and being published in English. Citations not meeting all of these criteria were excluded from review. All citations were screened and reviewed for inclusion by the author (D.I.). The initial search yielded 376 citations. 357 of these were excluded after reviewing titles and abstracts (310 for not mentioning neurobiological considerations in exercise, 27 for not relating to physical exercise, 17 for exclusively focusing on children, and 3 for not being published in English). Full texts of the remaining 19 citations were reviewed, with 4 of these articles excluded (for not clearly describing neurobiological aspects of exercise in adults). Reference lists of the remaining 15 articles were reviewed and led to identification of an additional 10 citations, for a total of 25 articles identified as relevant to the neurobiology of exercise in adults.
Figure 2 presents a schematic of the search process used to review the literature on the neurobiology of ASD in regard to repetitive, selfinjurious, and aggressive behavior. A search of the databases PubMed and Ovid MEDLINE from 1987 to 2024 was performed on March 12, 2024, using the search terms, “autism spectrum disorder,” “autistic,” “neurobiology,” “repetitive,” “stereotypy,” “stereotyped,”“self injurious,” “self injury,” and “aggression.” Inclusion criteria included needing to involve individuals with ASD, needing to describe neurobiological considerations in regard to repetitive, self-injurious, or aggressive behavior in ASD, and being published in English. Citations not meeting all of these criteria were excluded from review. All citations were screened and reviewed for inclusion by the author (D.I.). The initial search yielded 236 citations. 203 of these were excluded after reviewing titles and abstracts (187 for not clearly addressing the neurobiology of repetitive, self-injurious, or aggressive behavior in ASD, 14 for not clearly involving individuals with ASD, and 2 for not being published in English). Full texts of the remaining 33 articles were reviewed, with 7 of these articles excluded (for not clearly describing neurobiological underpinnings of repetitive, self-injurious, and/or aggressive behavior in ASD). Reference lists of the remaining 26 articles were reviewed and led to identification of an additional 14 citations, for a total of 40 articles identified as relevant to the neurobiology of ASD in regard to repetitive, self-injurious, or aggressive behavior.
Figure 2: Schematic of search process for reviewing neurobiology of autism spectrum disorder.
25 articles were identified that were assessed as relevant to the neurobiology of exercise in adults; [26-50] these are presented in Table 1. 40 articles were identified that were deemed relevant to the neurobiology of ASD in regard to repetitive, self-injurious, or aggressive behavior; [50-89] these are presented in Table 2.
Author (Year) | Study Type | Study Design | Sample Size | Findings | Study Quality |
Dishman RK, et al. [26] | Animal and human studies referenced | Synthesis/ commentary | Not applicable |
|
Unclear; no search process or assessment of quality outlined for studies referenced (commentary piece) |
Portugal EMM, et al. [27] | Animal and human studies referenced | Review (not clearly systematic) | Not applicable |
|
Medium; no search process or assessment of quality outlined for studies referenced, but detailed description of exercise and its impact on multiple neurobiological systems presented |
Meeusen R, et al. [28] | Animal studies referenced | Synthesis/ commentary | Not applicable |
|
Medium; no search process or assessment of quality outlined for studies referenced, but detailed description of microdialysis use in understanding impact of exercise on neurotransmission presented |
Dietrich A, et al [29] | Animal and human studies referenced | Review (not clearly systematic) | Not applicable |
|
Medium; no search process or assessment of quality outlined for studies referenced, but detailed description of cannabinoid system’s psychoactive, analgesic, and motor effects provided |
Iqbal M, et al. [30] | Animal study | Experimental (examined impact of physical training on hippocampal neurogenesis in mice who had experienced status epilepticus) | Unspecified |
|
Medium; while study had control group, well- described methods, and thoroughly presented results, no description of blinding of raters |
Bicker F, et al. [31] | Animal and human studies referenced | Review (not clearly systematic) | Not applicable |
|
Medium; no search process or assessment of quality outlined for studies referenced, but detailed description of adult neurogenesis molecular pathways and relation to ASD provided |
Saraulli D, et al. [32] | Animal and human studies referenced | Review (not clearly systematic) | Not applicable |
|
Medium; no search process or assessment of quality outlined for studies referenced, but detailed description of neuroprotective role of physical activity across life stages presented |
Grońska PM, et al. [33] | Animal study | Experimental (examined impact of FGFR gene deletions in mice on enriched environment (including exercise)-induced hippocampal neurogenesis) | Unspecified |
|
High; study employed control group, well- described methods, thoroughly presented results, and blinding of raters |
Hendrikse J, et al. [34] | Human study | Experimental (between- subjects, cross- sectional design; examined associations between exercise/ cardiorespiratory fitness and hippocampal structure and function) | N=40 (healthy adults, mean age=25.48 years, 52.5% female) |
|
Medium; well-described methods, reasonably complete result reporting, attempts made to minimize confounding variables/ selection bias; however, no clear blinding of raters, and data from 2 subjects who dropped out of study not included in analysis |
Ledreux A, et al. [35] | Human study | Experimental (compared impact of 5 weeks of exercise, cognitive training, or mindfulness practice on serum BDNF levels in healthy older adults) | N=146 (mean age 72.9 years, 69.2% female) |
|
High; large sample size, use of controls, randomization procedure described; however, unclear blinding and BDNF levels drawn nearly 4 days after last exercise session, which may have affected results |
Voss MW, et al. [36] | Human study | Experimental (examined impact of aerobic vs non- aerobic exercise on brain function and cognition, including functional connectivity between higher cognitive networks) | N=65 (mean age 66.34 years, 72% female) |
|
Medium; well-described methods, reasonably complete result reporting, attempts made to minimize confounding variables/ selection bias; however, randomization procedure, allocation concealment, and rater blinding not described |
Voss MW, et al. [37] | Human study | Experimental (examined impact of 1-year aerobic exercise program for healthy older adults on serum levels of BDNF, IGF-1, and VEGF, and whether these levels correlated with changes in functional connectivity, compared to non- aerobic control group) | N=65 (mean age=66.35 years, 72% female) |
|
Medium; well described/ thoroughly presented methods and results, attempts made to minimize confounding variables/ selection bias; however, randomization procedure, allocation concealment, and rater blinding not described |
Kronman CA, et al. [38] | Human study | Experimental (examined whether cardiorespiratory fitness predicts effective connectivity between HC and other DMN nodes in healthy young adults) | N=26 (mean age 26 years, 64% female) |
|
Medium; well described/ thoroughly presented methods and results, attempts made to minimize confounding variables/ selection bias; however, randomization procedure, allocation concealment, and rater blinding not described |
Rimmele U, et al. [39] | Human study | Experimental (examined whether high physical activity level can modulate physiological and psychological responses to psychosocial stress) | N=44 (mean age 21.7 years, 0% female) |
|
High; experimental and comparison groups well-matched, objective measures of stress response, no selective reporting; nonrandomized study |
Drogos LL, et al. [40] | Human study | Experimental (examined effect of exercise on cortisol awakening response and subjective stress in sedentary but otherwise healthy older adults) | N=32 (mean age 65 years, 60% female) |
|
High; well described/ thoroughly presented methods and results; nonrandomized study with subjects serving as own controls |
Stranahan AM, et al. [41] | Animal studies referenced | Review (not clearly systematic) | Not applicable |
|
Medium; no search process or assessment of quality outlined for studies referenced, but detailed description of potential effects of exercise on stress- related systems (including HPA axis) provided |
de Souza RF, et al. [42] | Animal studies referenced | Systematic review and meta-analysis | Not applicable (27 studies included) |
|
High; PRISMA guidelines used; risk of bias tools used to evaluate quality of studies |
Duman CH, et al. [43] | Animal study | Experimental (characterized effects of chronic exercise in mice relevant to antidepressant actions) | Not applicable |
|
High; use of control group, experimenters blinded to genotype and treatment history, thoroughly presented methods and results |
Sun L, et al. [44] | Animal and human studies referenced | Review (not clearly systematic) | Not applicable |
|
Medium; no search process or assessment of quality outlined for studies referenced, but detailed description of effects of exercise on neurotrophic, metabolic, and other factors impacting neurogenesis |
Russo- Neustadt A, et al. [45] | Animal study | Experimental (examined impact of combined antidepressant treatment and exercise on BDNF expression in rat hippocampus) | Unspecified |
|
Medium; thoroughly presented methods and results, use of control group; no description of blinding of raters |
Deslandes AC, et al. [46] | Human study | Quasi- experimental (examined impact of adding exercise to antidepressant treatment in older adults with DSM- IV MDD; subjects could choose assignment) | N=20 (mean age 71 years, 70% female) |
|
Medium; thoroughly presented methods and results, use of control group; no randomization or description of blinding |
Song R, et al. [47] | Human studies referenced | Systematic review and meta-analysis | N=735 subjects from 21 studies (mean age 67.5 years, 41.5% female) |
|
High; PRISMA guidelines used; risk of bias tools used to evaluate quality of studies |
Hirsch MA, et al. [48] | Human study | Experimental (compared high-intensity resistance training + balance training to balance training alone in subjects with PD) | N=15 (mean age 73.25 years, gender ratio not specified) |
|
Medium; thoroughly presented methods and results, randomized, but no control group or description of blinding and sample size small |
Bloomer RJ, et al. [49] | Human study | Experimental (examined effect of exercise on oxidative status in subjects with PD) | N=16 (mean age 59 years, 50% female) |
|
Medium; well-described methods, results, and randomization method, use of control groups; however, no description of blinding and sample size small |
Sutoo D, et al. [50] | Animal studies referenced | Review (not clearly systematic) | Not applicable |
|
Unclear; no search process or assessment of quality outlined for studies referenced |
Table 1: Reviewed Studies on Neurobiology of Exercise in Adults.
ACTH: Adrenocorticotropic hormone; ASD: Autism Spectrum Disorder; BDNF: Brain Derived Neurotrophic Factor; CB1: Cannabinoid Receptor Type 1; CRH: Corticotropin Releasing Hormone; DSM-IV: Diagnostic and Statistical Manual of Mental Disorders, Fourth Edition; DMN: Default Mode Network; DMPFC, Dorsomedial Prefrontal Cortex; FGFR: Fibroblast Growth Factor Receptor; FEN: Frontal Executive Network; HC: Hippocampus; HPA: Hypothalamic-Pituitary-Adrenal; IGF, Insulin-Like Growth Factor; IGF-1: Insulin-Like Growth Factor Type 1; MDD: Major Depressive Disorder; MRI: Magnetic Resonance Imaging; mRNA: Messenger Ribonucleic Acid; MRS: Magnetic Resonance Spectroscopy; PD: Parkinson’s Disease; PET: Positron Emission Tomography; PFC: Prefrontal Cortex; PRISMA: Preferred Reporting Items for Systematic Reviews and Meta-Analyses; PVN: Paraventricular Nucleus; VEGF: Vascular Endothelial Growth Factor; VMPFC: Ventromedial Prefrontal Cortex
Author (Year) | Study Type | Study Design | Sample Size | Findings | Study Quality |
Kemper TL, et al. [51] | Human study (post-mortem analyis) | Observational/post- mortem analysis of brains of individuals who had diagnoses of ASD | N=6 (age range 9 to 29 years: 16.67% female) |
|
Medium; well-described methods and results, control brains provided, but small sample size |
Kemper TL, et al. [52] | Human study (post-mortem analysis) | Observational/post- mortem analysis of brains of individuals who had diagnoses of ASD | N=9 (age range not specified: unclear gender distribution) |
|
Medium; well-described methods and results, control brains matched for age and sex and identically processed, but small sample size |
Bailey A, et al. [53] | Human study (post-mortem analysis) | Observational/post- mortem analysis of brains of individuals who had diagnoses of ASD | N=6 (age range 4 to 27 years: 0% female) |
|
Medium; well-descrbed methods and results, control brains matched for age and identically processed, but small sample size |
Aylward EH, et al. [54] | Human study | Case-control design comparing amydala, hippocampal, and total brain volumes on MRI between individuals (male adolescents and young adults) with and without ASD | N=28 (0% female) |
|
Medium; well-described methods and results, reasonable sample size, use of matched controls, but unclear if blinding applied to radiograph interpreters |
Abell F, et al. [55] | Human study | Case-control design comparing voxel-based whole-brain MRI scans between individuals with and without ASD | N=30 (mean age 26.5 years: 20% female) |
|
Medium; well-described methods and results, reasonable sample size, well-matched controls, ASD dxs carefully confirmed, but unclear if blinding applied to radiograph interpreters |
Tuchman R, et al. [56] | Human studies referenced | Review (not clearly systematic) | Not applicable |
|
Unclear; no search process or assessment of quality outlined for studies referenced |
Martella G, et al. [57] | Animal study | Case-control (examined expression of long- term synaptic plasticity at corticostriatal glutamatergic synapses in dorsal striatum of mice carrying mutation inNL3 gene compared to WT mice without mutation) | Unspecified |
|
Medium; well-described/ thoroughly presented methods and results, WT littermates used as controls, but no description of blinding of raters |
Green SA, et al. [58] | Human study | Experimental (examined differences in brain responses, habituation, and connectivity using fMRI during exposure to mildly aversive sensory stimuli in youth with ASD and SOR compared with youth with ASD without SOR and compared with typically developing control subjects) | N=38 (mean age 13.71 years: 16% female) |
|
Medium; well-described methods and results, reasonable sample size, well-matched controls, but unclear if blinding applied to radiograph interpreters |
Hazen EP, et al. [59] | Human studies referenced | Review (described as “systematic review”) | Not applicable |
|
Medium; search process outlined, but no assessment of quality of reviewed studies provided, and unclear if PRISMA guidelines followed for systematic reviews |
McCarty MJ, et al. [60] | Human studies referenced | Opinion piece/ commentary | Not applicable |
|
Low/not applicable (opinion/commentary piece; as such, no search process or assessment of quality outlined for studies referenced) |
Willsey AJ, et al [61] | Animal and human studies referenced | Review (not clearly systematic) | Not applicable |
|
Medium; no search process or assessment of quality outlined for studies referenced |
Castelli F, et al. [62] | Human study | Experimental (examined PET findings during mentalizing task in subjects with ASD compared to healthy controls) | N=10 (mean age 33 years in ASD group: 25 years in control group; gender distribution unspecified) |
|
High; well-described methods and results, well- matched controls (except control group slightly younger), blinding of raters adequately described; modest sample size |
Thompson L, et al. [63] | Human studies referenced | Case series (reports on individuals with ASD who had received quantitative EEG monitoring) | N= >150 subjects (age range and gender distribution unspecified) |
|
Medium; well-described methods and results, large sample size, well matched controls, but unclear if blinding applied to EEG interpreters |
Hwang BJ, et al. [64] | Human studies referenced | Review (not clearly systematic) of molecular (MRS) and nuclear (PET, SPECT) imaging findings in ASD | Not applicable |
|
Medium; while no search process or assessment of quality outlined for studies referenced, comprehensive list of definitions and descriptions provided for molecular and nuclear imaging techniques and associated findings in individuals with ASD |
Segura M, et al. [65] | Human study | Case-control (examined peripheral blood mRNA expression of NT3, NT4, and BDNF in subjects with ASD compared to healthy controls) | N=21 (mean age 19.7 years: 4.76% female) |
|
Medium; well-described methods and results, well-matched controls, but modest sample size and unclear if blinding of assessors employed |
Taurines R, et al. [66] | Human study | Case-control (examined serum concentrations and peripheral blood mRNA expression of BDNF in subjects with ASD compared to healthy controls) | N=24 for BDNF conc (mean age 13.9 years); N=16 for mRNA expression (mean age 10.8 years); all subjects male |
|
Medium; well-described methods and results, well-matched controls, but modest sample sizes and unclear if blinding of assessors employed |
Tostes MH, et al. [67] | Human study | Case-control (compared plasma levels of VIP, NT3, cytokines, and NO in children with ASD to age- and gender- matched healthy controls | N=24 |
|
Medium; well-described methods and results, well- matched controls, but modest sample sizes and unclear use of blinding |
Bjorklund G, et al. [68] | Human studies referenced | Review (not clearly systematic) | Not applicable |
|
Medium; no search process or assessment of quality described for studies referenced, although comprehensive description of oxidative stress markers provided |
Chauhan A, et al. [69] | Human study | Case-control (compared lipid peroxidation status and serum levels of antioxidant proteins (transferrin and ceruloplasmin) in children with ASD to their siblings without ASD) | N=19 for lipid perox (mean age 4.4 years); N=11 for antioxidant proteins (mean age 6.0 years); gender distribution unspecified |
|
Medium; well-described methods and results, well-matched controls, but modest sample sizes and unclear if blinding of assessors employed |
Baker EK, et al. [70] | Human study | Case-control (compared sleep, arousal, and cortisol responses among adults with ASD (medicated for anxiety and/or depression and non-medicated) and controls without ASD) | N=29 (age range 21 to 44 years) |
|
Medium; well-described methods and results, well-matched controls, reasonable sample size; unclear if blinding of assessors employed |
Edmiston EK, et al. [71] | Human study | Case-control (compared salivary cortisol response to social evaluative threat in adolescents with ASD to controls without ASD) | N=28 (mean age 14.8 years: 14% female) |
|
Medium; well-described methods and results, well-matched controls, reasonable sample size; unclear if blinding of assessors employed |
Corbett BA, et al. [72] | Human study | Case-control (compared circadian rhythms and salivary cortisol response to a novel stimulus in children with ASD to controls without ASD) | N=22 (mean age 8.81 years: 4.5% female) |
|
Low; well-described methods and results, but poorly matched controls (e.g., IQ significantly lower in ASD group), modest sample size, unclear blinding |
Kalueff AV, et al. [73] | Animal studies referenced | Review (not clearly systematic) | Not applicable |
|
Medium; no search process or assessment of quality described for studies referenced, although application of rodent self-grooming to understanding of various conditions provided |
Chmielewski WX, et al. [74] | Human studies referenced | Review (not clearly systematic) | Not applicable |
|
Medium; no search process or assessment of quality described for studies referenced, but detailed review of studies relevant to ASD action control processes presented |
Peca J, et al. [75] | Animal study | Case-control (examined impact of Shank3 gene deletions on self- injurious: repetitive grooming behavior and social interaction in mice compared to WT mice without the deletion) | Unspecified |
|
High; well-described methods and results, use of controls, description of blinding of all experimenters to genotype included |
Frackowiak J, et al. [76] | Human studies referenced | Theoretical | Not applicable |
|
Medium; no search process or assessment of quality described for studies referenced, but detailed review of studies relevant to Aβ accumulation hypothesis in ASD presented |
Muehlmann AM, et al. [77] | Animal and human studies referenced | Review (not clearly systematic) | Not applicable |
|
Medium; no search process or assessment of quality described for studies referenced, but detailed review of studies relevant to neurobiology of repetitive and SIB presented |
Yoon SY, et al. [78] | Animal study | Experimental (examined impact of intrathecal injection of NMDA on nociceptive behaviors in mice with and without Shank3 gene deletion) | Unspecified |
|
Medium; well-described methods and results, use of WT controls; unclear if blinding of assessors employed |
Farmer Al, et al. [79] | Animal studies referenced | Review (not clearly systematic) | Not applicable |
|
Medium; no search process or assessment of quality described for studies referenced, but detailed review of studies relevant to neurobiology of EEs and their potential impact on repetitive and SIB presented |
Bortolato M, et al. [80] | Animal studies referenced | Review (not clearly systematic) | Not applicable |
|
Medium; no search process or assessment of quality described for studies referenced |
Wu Y, et al. [81] | Human studies referenced | Systematic review of safety and efficiacy of stereotactic neurosurgery for ASD | N=36 (mean age 25.64 years: 19.44% female) |
|
Medium; clear description of inclusion/exclusion criteria, search process, included studies; however, most studies were case reports, data incomplete for several studies, several studies did not note whether ASD dx based on standardized diagnostic criteria |
Gouveia FV, et al. [82] | Human study | Case-control (examined cortical thickness in individuals with ASD with treatment- refractory aggressive behavior [rAB] compared to those with ASD without refractory aggressive behavior [nrAB]) | N=10 (age ranges 19 to 29 years for rAB group: 11 to 24 years for nrAB group; 0% female) |
|
Medium; well-described methods and results (including use of validated scales to measure aggression), but modest sample size and unclear if blinding of MRI image interpreters employed |
Rajamani KT, et al. [83] | Human studies referenced | Review (not clearly systematic) | Not applicable |
|
Medium; no search process or assessment of quality described for studies referenced, although detailed description of oxytocin’s role in six genetic syndromes associated with ASD well described |
Burrows EL, et al. [84] | Animal study | Experimental (examined level of aggression in response to juvenile mouse intruder in mice with R451C mutation in NL3 gene compared to WT control mice; also examined occurrence of repetitive behavior in R451C NL3 mutant mice) | Unspecified |
|
High; well-described methods and results, use of WT controls, clear blinding of experimenter to genotype and exact sample sizes |
Strekalova T, et al. [85] | Animal study | Experimental (examined level of attacking and other abnormal behaviors in response to various behavioral tests in mice with St3gal5 mutation compared to WT control mice) | Unspecified |
|
Medium; well-described methods and results, use of WT controls, but unclear blinding of experimenter to genotype |
Armstrong EC, et al. [86] | Animal study | Experimental (examined level of aggressive and other behaviors in response to behavioral tests in
mice with Neurexin 1![]() |
Unspecfied |
|
Medium; well-described methods and results, use of WT controls, but unclear blinding of experimenter to genotype |
Brodkin ES [87] | Animal studies referenced | Review (not clearly systematic) | Not applicable |
|
Medium; search strategy clearly outlined, detailed description of ASD-related phenotypes in BALB/c mice provided; however, no clear assessment of quality of included studies |
Fazel Darbandi S, et al. [88] | Animal study | Case-control (examined impact of Tbr1 deletion in mice related to cortical 6 layer neurons and behavioral phenotypes compared to WT control mice) | Unspecified |
|
Medium; well-described methods and results, use of WT controls, but unclear blinding of experimenter to genotype |
Markopoulos A, et al. [89] | Human studies referenced | Review (not clearly systematic) | Not applicable |
|
Medium; no search process or assessment of quality described for studies referenced, although detailed review of studies examining psychedelic use in non- ASD subjects presented |
Rojas-Charry L, et al. [90] | Animal studies reviewed | Review (not clearly systematic) | Not applicable |
|
Medium; no search process or assessment of quality described for studies referenced, but detailed review of studies examining mitochondrial disturbances in specific brain regions in ASD presented |
Table 2: Reviewed Studies on Neurobiology of Autism Spectrum Disorder.
Aβ: Amyloid Beta; AC: Anterior Cingulate; ACC: Anterior Cingulate Cortex; ASD: Autism Spectrum Disorder; BDNF: Brain Derived Neurotrophic Factor; CB1: Cannabinoid Receptor Type 1; Conc: Concentration; DLPFC: Dorsolateral Prefrontal Cortex; EE: Enriched Environment; EEG: Electroencephalography; fMRI: Functional Magnetic Resonance Imaging; GABA: Gaba-Amino-Butyric acid; HC: Hippocampus; HCG: Hippocampal Gyrus; 5-HT: 5-Hydroxytryptamine; HPA: Hypothalamic-Pituitary-Adrenal; IFN-γ: Interferon-Gamma; IQ: Intelligence Quotient; MAO-A: monoamine oxidase A; MPFC: Medial Prefrontal Cortex; MRI: Magnetic Resonance Imaging; mRNA: Messenger Ribonucleic Acid; MRS: Magnetic Resonance Spectroscopy; NL3: Neuroligin 3; NL4: Neuroligin 4; NMDA: N-Methyl-D-Aspartate; NO: Nitric Oxide; nrAB: Non-Refractory Aggressive Behavior; OAS: Overt Aggression Scale; OFC: Orbitofrontal Cortex; PHCG: Parahippocampal Gyrus; Perox: Peroxidation; PET: Positron Emission Tomography; PFC: Prefrontal Cortex; rAB: Refractory Aggressive Behavior; R451C: Arginine-To-Cysteine Residue 451 Substitution; SIB: Self-Injurious Behavior; SOR: Sensory Over Responsivity; SERT: Serotonin Transporter; SNPR: Substantia Nigra Pars Reticulata; SPECT: Single Photon Emission Computed Tomography; STS: Superior Temporal Sulcus; VIP: Vasoactive Intestinal Peptide; WT: Wild Type; YBOCS: Yale Brown Obsessive Compulsive Scale
While a uniform approach to assessing the quality of the identified studies was challenging due to the wide variety of publications reviewed (including animal studies, human studies, commentaries, general [non-systematic] reviews, systematic reviews, observational [post-mortem] studies, case-control studies, quasi-experimental designs, and experimental designs), an attempt was made to assess study quality for each article by incorporating relevent elements from the Cochrane Collaboration’s tool for assessing risk of bias in randomized trials, [90] Cochrane ROBINS-I (Risk of Bias in Nonrandomized Studies of Interventions) tool for assessing risk of bias in nonrandomized treatment studies, [91] and Reichow tool for assessing risk of bias in single-case research design [92].These elements, along with the author’s judgment in cases of commentaries and reviews, were used to assign a rating of “low,” “medium,” “high,” or “unclear” to the quality of each article, as shown in the last column of Tables 1 and 2.
To explore possible mechanisms by which exercise may alleviate repetitive, self-injurious, and aggressive behaviors in adults with ASD, the neurobiology of exercise was reviewed first.
Neurobiology of exercise
The neurobiology of physical exercise has received increasing attention over the last 15 years, [26,27] with studies revealing a variety of neurobiological responses to exercise.
Animal studies have shown that acutely, exercise modulates the central nervous system (CNS) neurotransmitters serotonin, norepinephrine, and dopamine by increasing their extracellular concentrations in the striatum, hippocampus, and frontal cortex, with the intensity and duration of exercise correlated with levels of neurotransmitter release [28]. Exercise has also been shown to be associated with the release of endogenous opioids and endocannabinoids, which can produce euphoric, anxiolytic, sedative, and analgesic effects in humans [29]. In addition to affecting neurotransmitters/neurochemicals, exercise has been shown in animal studies to activate the expression of neurotrophic factors, including Brain-Derived Neurotrophic Factor (BDNF), insulin-like growth factor (IGF-1), Vascular Endothelial Growth Factor (VEGF), Neurotrophin-3 (NT3), and Neurotrophin-4 (NT4) [27]. These factors serve to promote survival, proliferation, and maturation of specific brain cells (such as those of the hippocampus), activate signaling pathways involved in transcription [such as cAMP Response ElementBinding Protein (CREB)], and inhibit signals in apoptotic pathways (involved in neuronal cell death) [27,30].
Long-term, physical exercise has been shown to increase cerebral blood flow and to continue inducing expression of neurotrophic factors, [31] resulting in enhanced neurogenesis (BDNF and IGF-1), angiogenesis (VEGF), and synaptogenesis (BDNF) [32]. For example, exercise has been found to increase the proliferation of progenitor cells as well as the length, arborization, and spine density of newly formed granule cells in the dentate gyrus of the hippocampus, a region that mediates learning and memory formation, suggesting that exercise may increase newborn cells and improve synaptic plasticity within this network [32-34]. Despite these positive findings, not all studies have demonstrated increased serum BDNF levels from physical exercise. For example, Ledreux A, et al. [35]found that after 5 weeks, cognitive training, but not exercise or mindfulness practice, was associated with increased serum BDNF levels in healthy older adults, although there was a non-significant trend toward exercise-associated increased serum BDNF levels in one of two cohorts, and serum BDNF levels were drawn on average 3.8 days after the last exercise session, which could explain the lack of observed exercise effect.
Physical exercise has also been shown to improve functional connectivity within higher-level cognitive networks [36-38]. According to Voss MW, et al. [37], from a functional Magnetic Resonance Imaging (fMRI) standpoint, functional connectivity refers to a measure of the temporal coherence between spatially remote brain regions, such that two regions with a positive correlation in signal over time are said to have high functional connectivity, whereas regions uncorrelated or negatively correlated are thought to be in separate brain networks. These researchers note that the Default Mode Network (DMN) includes the posterior cingulate, medial frontal, bilateral occipital, middle temporal, hippocampal, and parahippocampal cortices, and is involved in memory consolidation, self-referential thought, and mind wandering, with greater metabolic activity in this network demonstrated during theory of mind processes (i.e. those requiring a person to identify and appreciate the thoughts, feelings, and intentions of others). The Frontal Executive (FE) network includes the anterior prefrontal cortex, insular and frontal operculum cortices, temporoparietal junction, and dorsal posterior and anterior cingulate gyri, with its roles to include sustaining attention for tasks, regulating top-down control (i.e. refining the ability of prefrontal and other higher cortical brain regions to modulate output from subcortical regions such the amygdala), and maintaining associations between actions and their outcomes. These researchers found that one year of a randomized aerobic exercise program (walking) improved functional connectivity in the DMN and FE networks in 65 healthy older adults compared to a non-aerobic control group, and that these changes correlated with increased BDNF, IGF-1 and VEGF [36].
Exercise has also been shown to affect the reactivity of the Hypothalamic-Pituitary-Adrenal (HPA) axis - critically involved in the human stress response - as exemplified by studies showing that subjects who had undergone physical exercise training demonstrated lower levels of cortisol in response to a stressor than sedentary subjects, [39] and that aerobic exercise improved HPA axis functioning (as measured by enhanced Cortisol Awakening Response) and decreased perceived stress in healthy older adults [40]. It has been hypothesized that biological changes in the activity of the HPA axis in response to exercise (e.g., enhanced density and efficiency of mineralocorticoid receptors, lower cortisol levels, inhibition of cortisol synthesis) could be due to a more efficient negative feedback mechanism induced by exercise, in which exercise-induced decreases in Corticotropin Releasing Hormone (CRH) mRNA transcription in the paraventricular nucleus of the hypothalamus could result in decreased ACTH release from the anterior pituitary and consequent lower levels of cortisol synthesis and release from the adrenal gland [41].
Long-term exercise has also been associated with anti-oxidant effects, [42] which could be explained by Reactive Oxygen Species (ROS)-mediated signaling. Specifically, mitochondrial production of ROS resulting from a high metabolic demand may induce signaling leading to the expression of genes encoding anti-oxidant enzymes (such as superoxide dismutase, catalase, and glutathione peroxidase) that counter the accumulation of free radicals. Indeed, exercise periods of longer than eight weeks have been associated with increases in superoxide dismutase and catalase activity in rodents [42]. In addition, an increased concentration of ROS may modulate intracellular pathways involving the CREB protein and the peroxisome proliferator-activated receptor-ɣ coactivator (PGC-1α) in the nucleus, inducing mitochondrial biogenesis. Hence, chronic exercise may induce both anti-oxidant and mitochondrial biogenic activity [27].
A number of neurophysiological studies have shown positive effects of exercise on the alleviation of depressive symptoms [43-46]. For example, studies in mice suggest that exercise-induced expression of hippocampal BDNF may account for antidepressant effects of physical exercise, supported by observations that BDNF-knockout mice show impaired antidepressant response and that BDNF infusion or the over-expression of TrkB receptors (receptors that bind BDNF) in the hippocampus induces a strong antidepressant-like response [43]. Moreover, in one animal study, [45] a combination of regular exercise and antidepressant treatment with imipramine or tranylcypromine produced a larger increase in the expression of BDNF mRNA in the dentate gyrus of the hippocampus than either intervention alone, suggesting that exercise may enhance treatment response to antidepressants for depression. Other studies suggest that exerciseinduced release of neurotransmitters and increase in neurotrophin activity may contribute to both neuroplasticity and unsuppressed cortical activity, which can improve depressive symptoms [46].
Exercise has also been studied in the treatment of neurodegenerative diseases such as dementia and Parkinson’s Disease [47]. These studies have revealed that regular physical exercise reduces amyloidbeta (Aβ) deposition, helps to prevent further brain atrophy and temporal lobe volume loss, and, in animal studies, induces hippocampal neurogenesis while causing significant improvements in hippocampus-dependent learning and memory [47]. Exercise has also been shown to improve motor functioning, quality of life, and control of body stability, [47,48] and to reduce serum levels of oxidative stress biomarkers in Parkinson’s Disease [49]. It has been postulated that sustained improvements in body stability following exercise may be due to increased neurogenesis, mitochondrial activity, and synthesis of certain neurotransmitters, such as dopamine [50]. Thus, increased neurotransmitter and neurotrophic factor activation along with antioxidant and mitochondrial biogenic effects appear to underlie the positive effects of exercise on neurodegenerative diseases such as dementia and Parkinson’s disease.
In summary, available data to date indicate that the neurobiology of physical exercise is characterized by modulatory effects on CNS neurotransmitters, neurotrophic factors, functional connectivity within higher-level cognitive networks, the HPA axis, and oxidative stress, resulting in increased neurogenesis, angiogenesis, synaptogenesis, ability to manage stress, and neuronal resilience. These effects are supported by neuroscientific findings (e.g., PET, functional MRI studies) showing, for example, increased hippocampal volumes in response to regular exercise, and clinical and epidemiologic data showing improvements in mood, cognition (e.g. learning and memory), and motor skills with exercise. Next, the neurobiology of ASD was reviewed.
Neurobiology of ASD
Evidence suggests that specific brain regions and the interconnections between them may play a role in causing the difficulties in social cognition, emotion regulation, and repetitive behaviors that typify individuals with ASD [51-56]. For example, neuropathologic studies in ASD have revealed smaller, more densely packed neurons in the amygdala, hippocampus, entorhinal cortex, mammillary body, anterior cingulate gyrus, and nuclei of the septum; [51,52] decreased numbers of Purkinje cells in the posterolateral and inferior cerebellum; [52,53] and cortical abnormalities [53]. Morphometric studies have noted volumetric reductions in the amygdala and hippocampus relative to total brain volume, and decreases in gray matter in the right paracingulate gyrus and left inferior frontal gyrus in patients with ASD compared with control patients; [54,55] according to Aylward EH, et al. [54] these findings (along with the aforementioned histopathologic observations) suggest dendritic tree and neuropil underdevelopment, reflecting incompletely developed connections between limbic system structures and the cerebral cortex.
Martella G, et al. [57] studied the expression of long-term synaptic plasticity at corticostriatal glutamatergic synapses in the dorsal striatum of mice carrying a mutation in the neuroligin 3 (NL3) gene, a gene that has been associated with highly penetrant ASD in a Swedish family. These researchers found that the expression of longterm synaptic depression at glutamatergic synapses in this region was impaired by the mutation, but that this depression was partially reinstated by exogenous activation of pre-synaptic cannabinoid CB1 or post-synaptic endocannabinoid receptors. They concluded that the dorsal striatum is substantially implicated in ASD pathophysiology, that an altered cannabinoid drive may underlie deficient synaptic plasticity in this region, and that activation of the endocannabinoid system may exert beneficial effects. They noted that the striatum’s role in ASD is supported by studies demonstrating that striatal neurons show enriched expression of genes associated with ASD, that brain imaging studies show abnormal striatal development in individuals with ASD from late childhood to early adulthood, that normal activation of the striatum in response to a social reward is lost in ASD, and that striatal dopamine and acetylcholine have been shown to be crucial for the induction and blockade of stereotyped behaviors, a key feature of ASD.
Green SA, et al., [58] using functional MRI, found that youth with ASD and sensory over-responsivity showed sensorilimbic hyperresponsivity to mildly aversive tactile and auditory stimuli, particularly to multiple modalities presented at the same time, and that this overresponsivity was due to a failure to habituate to such stimuli. However, they found that youth with ASD without sensory over-responsivity were able to regulate their responses to sensory stimuli through prefrontal down-regulation of amygdala activity, suggesting that enhancing topdown regulation processes and minimizing simultaneous exposure to multiple sensory modalities may be helpful for individuals with ASD. Other researchers have posited that sensory dysfunction may underly repetitive and self-injurious behaviors in ASD, [59] and that stereotypies may help individuals with ASD focus the mind and cope in overwhelming sensory environments by regulating brain rhythms either directly from the rhythmic motor command (motor cortex), or via rhythmic sensory feedback generated by the movements [60].
Willsey AJ, et al. [61] similarly noted that the prefrontal cortex, orbitofrontal cortex, and striatum are implicated in the neurobiology of ASD, based on developmentally informative studies of gene expression from early fetal to late adult stages. For example, they noted that areas particularly enriched in ASD candidate genes included deep layer (layers V-VI) cortical projection neurons in the midfetal prefrontal cortex, the prenatal orbitofrontal cortex, and the midfetal cortex and striatum.
Other research has similarly implicated involvement of prefrontal, cortical, and limbic regions and their interconnections in the neurobiology of ASD. For example, Positron Emission Tomography (PET) studies have shown, during theory-of-mind tasks, decreased activation in the prefrontal cortex, superior temporal sulcus at the temporo-parietal junction, and temporal poles, as well as reduced functional connectivity between the extrastriate cortex and superior temporal sulcus at the temporo-parietal junction in individuals with ASD, suggesting that a bottleneck in the interaction between higher order and lower order perceptual processes may account for the mentalizing deficits in ASD [62]. Moreover, quantitative electroencephalogram (EEG) studies have found significant differences between individuals with ASD and control subjects in the amplitude of slow and fast waves in the frontal, temporal, and temporalparietal cortices (so-called mirror neuron areas, i.e., areas containing visuomotor neurons that may have a role in imitation, empathy, understanding, and predicting others’ behavior). Such studies have also revealed abnormalities in the anterior cingulate, amygdala, uncus, insula, hippocampal gyrus, parahippocampal gyrus, fusiform gyrus, and the orbitofrontal and ventromedial areas of the prefrontal cortex in ASD [63].
On a neurotransmitter level, PET studies have shown that children with ASD synthesize serotonin at a significantly slower rate than typically developing children; that men with ASD demonstrate reduced serotonin transporter binding in the anterior and posterior cingulate cortices (correlating with impairment in social cognition) and in the thalamus (correlating with repetitive and obsessive interests and behaviors); and that men with ASD may possess increased dopamine transporter binding in the orbitofrontal cortex [64]. Molecular imaging studies (using Magnetic Resonance Spectroscopy or MRS) have shown evidence of an imbalance between neuronal excitation produced by glutamate and neuronal inhibition produced by gamma-amino-butyric acid (GABA) in ASD [64]. For example, infants with ASD showed reduced sensorimotor GABA concentrations compared to matched typically developing infants, and these reduced GABA concentrations correlated with behavioral measures of inhibition.
Other research has suggested that abnormalities in the expression (or levels) of neurotrophic factors may play a role in ASD pathophysiology. For example, individuals with ASD have been found to have significantly lower peripheral blood mRNA expression of NT3, NT4, and BDNF [65,66]. Of note, NT3 and NT4 play an important role in the development of cerebellar Purkinje cells (PCs), with NT3 selectively increasing PC survival [65]. These cells serve as the primary efferent neurons of the cerebellar cortex, and their potential role in ASD has been suggested. Supporting this, as noted earlier, neuropathological studies have shown significant reductions in the number and size of PCs in ASD postmortem brain [52].
Additional research has suggested a role for oxidative stress in the neurobiology of ASD [67,68]. For example, individuals with ASD have been found to have elevated levels of Vasoactive Intestinal Peptide (VIP), a peptide that induces neuroprotection by causing the secretion of neurotrophic factors (e.g., NT3) and cytokines from astroglia; it has been posited that in ASD, there is a blockage in the VIP-induced production of neurotrophic factors, including NT3, leading to impaired development in multiple brain regions (e.g., hippocampus, cerebellar PCs), and that VIP-induced release of inflammatory cytokines (e.g., IFN-ɣ) may lead to increased oxidative stress, reflected in elevated IFN-ɣ and nitric oxide (NO) levels in subjects with ASD [67]. Also, individuals with ASD have been shown to have reduced levels of antioxidant enzymes such as superoxide dismutase and glutathione peroxidase, increased markers of lipid peroxidation (which is the main consequence of oxidative stress), and decreased levels of the antioxidant transport proteins transferrin and ceruloplasmin, with a positive correlation between reduced levels of these proteins and the degree of loss of previously acquired language skills [69].
Finally, a number of studies suggest that dysregulation of the hypothalamic-pituitary-adrenal (HPA) axis may be involved in the neurobiology of ASD. For example, Baker EK, et al. [70] found that, compared to adults with ASD who were medicated for anxiety and/or depression and control subjects without ASD, adults with ASD (who were not medicated) displayed dampened cortisol secretion in the evenings and mornings, suggestive of a dysregulated HPA axis. Other studies have shown that, in contrast to typically developing individuals, persons with ASD often do not show robust HPA axis activation in response to social evaluative threat [71]. Notably, as opposed to adults with ASD, children with ASD may show cortisol hyper- or variable secretion during the daytime and evening, [72] raising the possibility that the specific nature of HPA axis dysregulation in ASD may vary with developmental stage.
In terms of the neurobiology of specific behaviors associated with ASD, beginning with repetitive behaviors, animal and human models suggest that the striatum, basal ganglia, amygdala, hypothalamus, anterior cingulate cortex, and prefrontal cortex may be involved in the regulation of repetitive behaviors, consistent with the above-noted EEG and other studies [73,74]. For example, stimulation of glutamatergic neurons in the posterior dorsal portion of the medial nucleus of the amygdala has been shown to induce repetitive self-grooming in mice; [73] mice lacking the GABA-synthesizing enzyme glutamate decarboxylase 1 in striatal neurons similarly exhibit stereotypic grooming behavior; [73] and decreased fractional anisotropy in the white matter underlying the rostral anterior cingulate cortex and decreased cerebral blood flow in this structure have been shown to correlate with higher levels of repetitive behavior in humans with ASD [74].
Regarding self-injurious behavior, Peca J, et al. [75] found that mice with Shank3 gene deletions exhibit self-injurious repetitive grooming, and that cellular, electrophysiological, and biochemical analyses uncovered defects at striatal synapses and corticostriatal circuits in Shank3 mutant mice, suggesting that striatal synapses and corticostriatal circuits may be involved in repetitive and self-injurious behavior in ASD. As noted above, Hazen EP, et al. [59] posited that abnormalities in sensory function and their related neurobiology may underly repetitive and self-injurious behavior in ASD. Frankowiak J, et al. [76] proposed that the neurobiology of self-injurious behavior in ASD may include accumulation of amyloid beta (Aβ) peptides in cortical (GABAergic neurons) and subcortical structures that can affect (activate or repress) gene transcription, induce oxidative stress, activate and alter membrane receptor signaling, form calcium channels causing hyperactivation of neurons, and reduce GABAergic signaling, all causing disrupted function of synapses and neuronal networks. Muehlmann and Lewis [77], reviewed evidence that selfinjurious and repetitive behavior share overlapping pathophysiology, and noted that clinical and animal studies have found cortical basal ganglia circuitry dysfunction to mediate both. Yoon SY, et al. [78] investigated the relationship between Shank2 synaptic proteins and NMDA receptor function in ASD and concluded that altered pain sensitivity may contribute to self-injurious behavior in ASD, caused by mutations in Shank2 genes that suppress NMDA-mediated signaling in spinal pain transmission. Farmer AL, et al. [79] reviewed the literature on the relationship between self-injurious (and other repetitive) behaviors and the use of enriched environments (described later), and noted that reductions in such behaviors from the use of such environments in mice were associated with increased dendritic spine density, neuronal activation, and altered gene expression in the subthalamic nucleus; increased neuronal activation in the dorsolateral, dorsomedial, ventromedial, and ventrolateral striatum; increased neuronal activation in the globus pallidus, substantia nigra pars reticulata, frontal cortex, hippocampus, motor cortex, and nucleus accumbens; increased dendritic spine density in the dorsolateral striatum; increased BDNF in the striatum; and altered gene expression, increased BDNF, and increased TrkB-PLCy1-CaMKII pathway activation in the hippocampus and prefrontal cortex. In summary, the neurobiology of self-injurious behavior in ASD may involve defects in striatal synapses and corticostriatal circuits; abnormalities in brain structures and function related to sensory symptoms (e.g., prefrontal cortical top-down regulation of amygdalar output); accumulation of amyloid beta (Aβ) peptides in cortical GABAergic neurons and subcortical structures leading to functional disruption of synapses and neuronal networks; cortical basal ganglia circuitry dysfunction; pain hypersensitivity due to mutations in Shank2 genes that suppress NMDA-mediated signaling; and dysfunction in the hippocampus, nucleus accumbens, and motor cortex.
The neurobiology of aggression in ASD may involve monoamine oxidase deficiencies (particularly monoamine oxidase A); [80] abnormal amygdalar function; [81] morphometric abnormalities in cortical regions (including reduced mean whole-brain cortical thickness and increased local cortical thickness bilaterally in the dorsolateral prefrontal cortex, medial prefrontal cortex, anterior cingulate cortex, and intraparietal sulcus cortex, along with significantly decreased thickness in the right superior frontal gyrus and left middle temporal gyrus; [82] genetic mutations (including those related to oxytocin, [83] neuroligin-3 [NL3], [84] the ganglioside ST3Gal5, [85] neurexin 1α, [86] BALB/c, [87] and Tbr-1 [88] genes) leading to alterations in synaptic function (including synaptic activity and plasticity) and neuroimmune/inflammatory responses; abnormal serotonergic signaling; [87,89] abnormal prefrontal cortex activity; [89] and aberrant thalamocortical signaling [88,89]. Regarding amydalar factors, Wu, et al. [81] conducted a systematic review of the safety and efficacy of stereotactic neurosurgery (including deep brain stimulation and radiofrequency ablation) targeting the amygdala in 36 individuals (primarily adults) with ASD, and found that this intervention alleviated obsessive-compulsive and aggressive behavior symptoms, with a mean improvement of 42.74% and 59.59% in YaleBrown Obsessive Compulsive Scale [94] and Overt Aggression Scale [95] scores, respectively. These authors postulated that stereotactic neurosurgery targeting the amygdala alleviates aggression by reducing nucleus activity and thereby restoring homeostatic patterns in the brain. Regarding genetic factors, Burrows EL, et al. [84] studied aggression in mice using the ASD-associated R451C (arginine to cysteine residue 451 substitution) mutation in the neuroligin-3 (NL3) gene. They found a pronounced elevation in aggressive (and repetitive) behavior in NL3(R451C) mutant mice that was reversed by treatment with risperidone. Similarly, Armstrong EC, et al., [86] noting that mutations in the Neurexin 1α gene have been associated with deficits in excitatory synaptic function, examined the effects of Neurexin 1α deletion on behavior across a range of developmental time points and found that juvenile and adult male Neurexin 1α knock-out mice exhibited social deficits and increased levels of aggression; they concluded that the Neurexin 1α deletion results in behavioral alterations relevant to ASD across development.
In summary, neurobiological studies in individuals with ASD suggest structural and functional abnormalities involving the prefrontal cortex, frontal and temporal cortices, limbic system (including the amygdala, hippocampus, and anterior cingulate), striatum, cerebellum, and the interconnections between these areas as contributory to many of the symptoms of this disorder. In addition, abnormalities in neurotransmitter levels (including serotonin, dopamine, glutamate, and GABA), reduced expression/levels of neurotrophic factors, increased oxidative stress, and dysregulated HPA axis function are implicated in the neurobiology of ASD, with some of these factors (e.g., neurotrophin levels) likely moderating the aforementioned structural and functional brain region abnormalities. The neurobiology of specific behaviors related to ASD, including repetitive behavior, self-injury, and aggression, appears to involve abnormalities in many of these same systems, specifically the striatum, basal ganglia, amygdala, hypothalamus, and anterior cingulate cortex (repetitive behaviors); cortiostriatal circuits, GABAergic neurons in cortical and subcortical structures, cortical basal ganglia circuitry, pain pathways involving NMDA-mediated signaling, and the hippocampus, nucleus accumbens, motor cortex, and prefrontal cortex (self-injury); and aberrations involving the amygdala, prefrontal cortex, anterior cingulate cortex, intraparietal sulcus, superior frontal gyrus, lef middle temporal gyrus, genetic mutations, serotonergic signaling, prefrontal cortex activity, and thalamocortical signaling (aggression). Genetic mutations likely underlie at least some of these aberrations.
a) Exercise has been shown to modulate CNS neurotransmitters [26] that are dysregulated in individuals with ASD [64,96]. By normalizing the level and function of these neurotransmitters, exercise may theoretically help with repetitive, self-injurious, and aggressive behaviors in adults with ASD, to the extent that these neurotransmitters may mediate gene-environment interactions between exercise and specific brain areas like the hippocampus [96]. Potential support for this hypothesis comes from multiple controlled studies demonstrating reduction in these behaviors in adults with ASD with selective serotonin reuptake inhibitors [97,98] and dopamine antagonists [99].
b) Exercise has been shown to facilitate the release of endocannabinoids, [29] which may help reverse deficits in synaptic plasticity at corticostriatal glutamatergic synapses in the dorsal striatum, [57] an area that has been strongly implicated in the pathophysiology of ASD. This region, for example, has been associated with the induction and blockade of repetitive/stereotyped behaviors [57,75].
c) Exercise has been demonstrated to induce expression of a number of neurotrophic factors observed to be deficient in individuals with ASD [27,30-32,65]. These neurotrophins are important for neuronal cell growth, proliferation, and survival in specific brain regions, including the hippocampus - areas that have been associated with morphometric and neuropathologic abnormalities in ASD [52,54]. By increasing expression of these neurotrophins, exercise may in theory reverse the dendritic tree and neuropil underdevelopment characteristic of a number of brain regions in ASD, possibly translating into improvements in ASD-related symptoms and maladaptive behaviors [31].
d) Exercise has been shown to improve functional connectivity in higher cognitive networks responsible for processes documented to be deficient in ASD, such as theory of mind abilities (mediated by the Default Mode Network) and top-down control processes (mediated by the Frontal Executive network) [37,38]. By improving connectivity in these networks, exercise may improve the ability of individuals with ASD to recognize and appreciate the mental states of others, and enhance the ability of these individuals to demonstrate self-control over intense, emotionally-driven aggressive behaviors.
e) Exercise has been shown to modulate the reactivity of the HPA axis, [39,40] which has been shown to be abnormal in individuals with ASD [70] While studies suggest there may be variability in how this dysregulation manifests over the course of development in ASD, with studies suggesting cortisol hyper- or variable secretion in children/ adolescents [72] and hyposecretion in adults, [70] it is possible that exercise may enhance the efficiency of the negative feedback response of the HPA axis [41]. Moreover, the early development and persistence of anxiety in children with ASD may lead to over activation of the HPA axis, with subsequent burnout of the system by adulthood if anxiety remains untreated [100]. It is therefore possible that exercise may not only exert a direct beneficial effect on HPA axis function, but that by treating comorbid anxiety in individuals with ASD, exercise may help prevent HPA axis overactivation and subsequent burnout. A number of studies support beneficial effects of exercise on anxiety in children and adults [101,102].
f) Exercise, through its demonstrated anti-oxidant effects, may counter the oxidative stress that has been posited to contribute to the pathogenesis of ASD [42,67-69]. In addition, increased mitochondrial biogenic activity induced by exercise may further contribute to the positive effects of exercise on neurodegenerative diseases such as dementia and Parkinson’s disease [27]. It is reasonable to infer that the anti-oxidant and mitochondrial biogenic effects of exercise may counter neuronal deterioration and facilitate neurogenesis in ASD; supporting this, Rojas-Charry RL, et al. [90] noted that mitochondria influence calcium channel function at synapses, which regulate neurotransmitter release, and may play a role in Shank-3 proteinmediated changes in synaptic function and plasticity that may contribute to ASD.
g) Exercise has been demonstrated to improve symptoms of depression - a common comorbidity in adults with ASD - in both animal [44] and human [103] studies. It has been estimated that 37% of adults with ASD may have a lifetime prevalence of depressive disorder [104]. Therefore, by effectively treating comorbid depression, exercise has the potential to reduce impairment caused by both depression and behavioral difficulties in adults with ASD.
h) Exercise has been shown to reduce amyloid-beta deposition in studies of neurodegenerative diseases, [47] and such deposition in cortical GABAergic neurons and subcortical structures (leading to functional disruption of synapses and neuronal networks) has been posited to contribute to self-injurious behavior in ASD [76].
Table 3 presents a summary comparison of neurobiological aspects of exercise and ASD.
Exercise | Autism Spectrum Disorder |
Modulatory effects on CNS neurotransmitters (including serotonin, norepinephrine, dopamine) by increasing extracellular concentrations in striatum, hippocampus, and frontal cortex [28] | Slower serotonin synthesis (children); reduced serotonin transporter binding in anterior and posterior cingulate cortices in men (correlating with impairment in social cognition) and in the thalamus (correlating with repetitive and obsessive interests and behaviors) [64] Increased dopamine transporter binding in orbitofrontal cortex; [64] striatal dopamine and acetylcholine shown to be crucial for induction and blockade of stereotyped behaviors [57] Imbalance between neuronal excitation produced by glutamate and neuronal inhibition produced by GABA [64] |
Increased release of endogenous opioids and endocannabinoids, which can produce euphoric, anxiolytic, sedative and analgesic effects [29] | Altered cannabinoid drive may underlie deficient synaptic plasticity in dorsal striatum (substantially implicated in ASD pathophysiology), and activation of endocannabinoid system may exert beneficial effects [57] |
Induces expression of neurotrophic factors, [31] resulting in enhanced neurogenesis (BDNF and IGF-1), angiogenesis (VEGF) and synaptogenesis (BDNF) [32] | Abnormal expression/levels of neurotrophic factors, e.g., reduced peripheral blood mRNA expression of NT3, NT4, BDNF [65,66] |
Improved functional connectivity in higher cognitive networks including top-down control processes [36-38] | Sensory dysfunction may underly repetitive and self-injurious behaviors and may be related to deficient prefrontal cortical top-down regulation of amygdalar output [58] |
Modulates reactivity of HPA axis, possibly by enhancing efficiency of the negative feedback response [39-41] | Dysregulation of HPA axis, including dampened cortisol secretion in evenings and mornings, [70] lack of robust activation in response to social evaluative threat [71] |
Induces anti-oxidant and mitochondrial biogenic activity [27] leading to expression of genes encoding anti-oxidant enzymes (e.g., superoxide dismutase, catalase and glutathione peroxidase) that counter accumulation of free radicals | VIP-induced release of inflammatory cytokines (e.g., IFN-ɣ) may lead to increased oxidative stress, reflected in elevated IFN-ɣ and nitric oxide levels [67] Reduced levels of antioxidant enzymes (e.g., superoxide dismutase and glutathione peroxidase), increased markers of lipid peroxidation, decreased levels of antioxidant transport proteins [69] |
Reduces amyloid-beta (Aβ) deposition in neurodegenerative diseases such as dementia and Parkinson’s Disease [47] | Accumulation of amyloid beta (AB) peptides in cortical GABAergic neurons and subcortical structures leading to functional disruption of synapses and neuronal networks [76] |
Improves symptoms of depression in both animal [44] and human [103] studies | Depression common; 37% of adults with ASD may have lifetime prevalence of depressive disorder [104] |
BDNF: Brain Derived Neurotrophic Factor; CNS: Central Nervous System; GABA: Gamma-Aminobutyric Acid; HPA: Hypothalamic-Pituitary-Adrenal; IGF-1: Insulin-Like Growth Factor 1; IFN-ɣ: Interferon-Gamma; mRNA, messenger RNA; NT3: Neurotrophin 3; NT4: Neurotrophin 4; VEGF: Vascular Endothelial Growth Factor |
Table 3: Comparison of neurobiological aspects of exercise and autism spectrum disorder (ASD).
Although this paper has explored viable mechanisms by which physical exercise may reduce repetitive/stereotyped, self-injurious, and aggressive behavior in adults with ASD, there are a number of limitations to this analysis. First, the reviewed studies on the neurobiological effects of exercise were conducted in individuals without ASD; it is possible that although exercise in neurotypical (including older) adults affects many of the entities that are dysregulated in ASD (e.g., neurotransmitters, neurotrophic factors, higher cognitive networks, oxidative stress pathways), adults with ASD might possess unique genetic/biological characteristics that render these individuals less responsive to the beneficial effects of exercise compared to those without ASD. Second, there was significant heterogeneity in the studies reviewed regarding the type, frequency, intensity, and format (i.e. group, supervised, and individual) of exercise examined, making it difficult to conclude which exercise parameters would be most helpful in addressing repetitive, self-injurious and aggressive behavior in adults with ASD. Third, the purported effect of exercise on increasing expression of neurotrophic factors, while supported by most studies reviewed, was not supported in all studies [35]. Finally, brain studies, although potentially enlightening in proposing neuroanatomical and neurophysiological bases for behavior, do not necessarily correlate with behavior, and the meaning of specific brain differences between groups (e.g., individuals with ASD and individuals without ASD) requires further clarification.
As noted earlier, preliminary evidence in support of the ability of exercise to reduce repetitive, self-injurious, and aggressive behavior in adults with ASD can be found in the literature, and argue against the aforementioned possibility that adults with ASD are significantly less responsive to the beneficial effects of exercise than neurotypical adults, older individuals, or those with degenerative diseases. For example, Elliott Jr RO, et al. [23] studied six adults with ASD and moderate to profound intellectual disability in a controlled environment before and after exercise and non-exercise conditions, and found that vigorous, antecedent aerobic exercise (defined as exercise elevating heart rates to above 130 beats per minute after 20 minutes) via use of a motorized treadmill reduced aggression in one subject, reduced property destruction in one subject, decreased self-injurious behavior in another subject, and reduced maladaptive stereotypic behaviors (e.g., rocking, loud vocalizations, teeth grinding) in all six subjects.
Allison DB, et al. [24] using a modified A-B-A-B design, found that antecedent physical exercise (consisting of jogging resulting in heart rate elevations to 60-80% of maximum) reduced aggression toward others (including grabbing, hitting, kicking, scratching and biting) in a 24 year-old man with DSM-III-R-diagnosed Autistic Disorder [105] and severe intellectual disability; the authors found that exercise alone was more effective than a combination of exercise and lorazepam in reducing aggression.
Morrison H, et al., [25] studied the effects of exercise on four individuals with ASD, one of whom was a 21 year-old man with significant intellectual disability and communication deficits who exhibited self-injurious behavior that included biting his fingers to the point of tissue damage. These researchers extended previous research methodologically by conducting functional analyses of problem behaviors to verify that these behaviors were maintained by automatic reinforcement (i.e. by their sensory consequences), by using exercise activities identified by subject preference assessments, by evaluating the immediate and post-intervention effects of antecedent exercise, and by comparing exercise interventions to leisure item and social interaction interventions. They found that exercise reduced selfinjurious behavior both during and after the intervention compared to the leisure and social interaction interventions, and suggested that exercise may serve as an abolishing operation for self-injurious behavior in ASD, i.e. that it produces stimulation functionally similar to that produced by problem behavior and therefore may lead to diminution of such behavior.
Farmer AL, et al. [79] reviewed the literature regarding the relationship between environmental enrichment and restricted, repetitive behavior, and noted that envionmental enrichment (an experimental paradigm in which laboratory animals, typically rodents, are exposed to conditions which provide social, physical, and cognitive stimulation, including running wheels for physical exercise) may attenuate stereotypy through increased neurotrophic expression and concomitant increases in synaptic plasticity, particularly within the basal ganglia and indirect basal ganglia pathway.
These preliminary studies, while encouraging in terms of suggesting that exercise may reduce maladaptive stereotypic behaviors, selfinjurious behavior, and aggression in adults with ASD, highlight the need for additional research on the effects of exercise in adults with ASD. Such research would ideally examine a large sample of adults with ASD randomized to two conditions, a group receiving exercise as the primary intervention, and a control group (matched for age, gender, ASD severity, psychiatric comorbidity, and other variables) receiving attention or other non-exercise-based intervention. As in the Morrison H, et al. [25] study, functional analyses could be conducted on problem behaviors to ensure they were automatically reinforced, and exercise activities could be based on systematically conducted preference assessments. Inclusion criteria would include adults with ASD who displayed maladaptive stereotyped behaviors, self-injurious behavior, or aggression. Exclusion criteria could include adults with ASD who had recently received a newly initiated or recently adjusted intervention for ASD (e.g., pharmacotherapy for aggression, ABA for core ASD symptoms). Baseline and post-intervention measurements of neurobiological parameters such as peripheral neurotrophins, evening and morning salivary cortisol, inflammatory cytokines and other markers of oxidative stress, and functional neuroimaging studies would be helpful in further supporting or refuting the above proposed mechanisms for how exercise may affect maladaptive behaviors in adults with ASD.
In summary, this paper has attempted to explore possible mechanisms by which exercise may reduce maladaptive repetitive behavior, self-injurious behavior, and aggression in adults with ASD. Based on a comparison of the neurobiology of ASD and neurobiology of exercise, plausible mechanisms for an exercise-induced beneficial effect on the aforementioned problem behaviors include modulation of neurotransmitter release and function, facilitation of endocannabinoid release, increased expression of neurotrophic factors, improved functional connectivity in higher-level cognitive networks, reduced oxidative stress, improved HPA axis function, treatment of comorbid depression, and reduction of amyloid-beta deposition in cortical GABAergic neurons and subcortical structures. Future research should attempt to confirm or refute the efficacy of exercise in reducing maladaptive behaviors in ASD as well as the proposed mechanisms for such a relationship. Confirmation of these issues would have major ramifications in terms of revealing an evidence-based intervention for problematic behaviors in adults with ASD that is inexpensive, widely available, and far-reaching in terms of providing broad physical and mental health benefits for this understudied and underserved population.
- APA (2013) Diagnostic and statistical manual of mental disorders. 5th edition, American Psychiatric Association, Arlington, VA, USA.
- Dawson JE, Matson JL, Cherry KE (1998) An analysis of maladaptive behaviors in persons with autism, PDD-NOS, and mental retardation. Res Dev Disabil 19: 439-448. [Ref.]
- Carroll D, Hallett V, McDougle CJ, Aman MG, McCracken JT, et al. (2014) Examination of aggression and self-injury in children with autism spectrum disorders and serious behavioral problems. Child Adolesc Psychiatr Clin N Am 23: 57-72. [Ref.]
- Nahmias AS, Pellecchia M, Stahmer AC, Mandell DS (2019) Effectiveness of community-based early intervention for children with autism spectrum disorder: a meta-analysis. J Child Psychol Psychiatry 60: 1200-1209. [Ref.]
- Makrygianni MK, Gena A, Katoudi S, Galanis P (2018) The effectiveness of applied behavior analytic interventions for children with Autism Spectrum Disorder: A meta-analytic study. Res Autism Spectr Disord 51: 18-31. [Ref.]
- Howes OD, Rogdaki M, Findon JL, Wichers RH, Charman T, et al. (2018) Autism spectrum disorder: Consensus guidelines on assessment, treatment and research from the British Association for Psychopharmacology. J Psychopharmacol 32: 3-29. [Ref.]
- Grob CM, Lerman DC, Langlinais CA, Villante NK (2019) Assessing and teaching job-related social skills to adults with autism spectrum disorder. J Appl Behav Anal 52: 150-172.
- Oswald TM, Patel BW, Ruder S, Xing G, Stahmer A, et al. (2018) A Pilot Randomized Controlled Trial of the ACCESS Program: A Group Intervention to Improve Social, Adaptive Functioning, Stress Coping, and Self-Determination Outcomes in Young Adults with Autism Spectrum Disorder. J Autism Dev Disord 48: 1742-1760. [Ref.]
- Estes A, Munson J, Rogers SJ, Greenson J, Winter J, et al. (2015) Long-Term Outcomes of Early Intervention in 6-Year-Old Children With Autism Spectrum Disorder. J Am Acad Child Adolesc Psychiatry 54: 580-587. [Ref.]
- Peters-Scheffer N, Didden R, Korzilius H, Sturmey P (2011) A metaanalytic study on the effectiveness of comprehensive ABA-based early intervention programs for children with Autism Spectrum Disorders. Res Autism Spectr Disord 5: 60-69.
- Leaf JB, Leaf R, McEachin J, Cihon JH, Ferguson JL (2018) Advantages and Challenges of a Home- and Clinic-Based Model of Behavioral Intervention for Individuals Diagnosed with Autism Spectrum Disorder. J Autism Dev Disord 48: 2258-2266. [Ref.]
- Matson JL, Turygin NC, Beighley J, Rieske R, Turek K, et al. (2012) Applied behavior analysis in autism spectrum disorders: Recent developments, strengths, and pitfalls. Res Autism Spectr Disord 6: 144-150.
- Dillenburger K, Keenan M, Doherty A, Byrne T, Gallagher S (2012) ABA-based programs for children diagnosed with autism spectrum disorder: Parental and professional experiences at school and at home. ChildF am Behav Ther 34: 111-129.
- Wink LK, Early M, Schaefer T, Pottenger A, Horn P, et al. (2014) Body mass index change in autism spectrum disorders: comparison of treatment with risperidone and aripiprazole. J Child Adolesc Psychopharmacol 24: 78-82. [Ref.]
- Ward SC, Whalon K, Rusnak K, Wendell K, Paschall N (2013) The association between therapeutic horseback riding and the social communication and sensory reactions of children with autism. J Autism Dev Disord 43: 2190-2198. [Ref.]
- Movahedi A, Bahrami F, Marandi SM, Abedi A (2013) Improvement in social dysfunction of children with autism spectrum disorder following long term Kata techniques training. Res Autism Spectr Disord 7: 1054-1061.
- Oriel KN, George CL, Peckus R, Semon A (2011) The effects of aerobic exercise on academic engagement in young children with autism spectrum disorder. Pediatr Phys Ther 23: 187-193. [Ref.]
- Hanley CA, Tureck K, Schneiderman RL (2011) Autism and exergaming: effects on repetitive behaviors and cognition. Psychol Res Behav Manag 4: 129-137. [Ref.]
- Ferreira JP, Ghiarone T, Júnior CRC, Furtado GE, Carvalho HM, et al. (2019) Effects of Physical Exercise on the Stereotyped Behavior of Children with Autism Spectrum Disorders. Medicina (Kaunas) 55: 685. [Ref.]
- Tarr CW, Rineer-Hershey A, Larwin L (2020) The effects of physical exercise on stereotypic behaviors in autism: small-n meta-analyses. Focus Autism Other Dev Disabl 35:26-35.
- Tse CYA, Pang CL, Lee PH (2018) Choosing an Appropriate Physical Exercise to Reduce Stereotypic Behavior in Children with Autism Spectrum Disorders: A Non-randomized Crossover Study. J Autism Dev Disord 48: 1666-1672. [Ref.]
- Shahane V, Kilyk A, Srinivasan SM (2024) Effects of physical activity and exercise-based interventions in young adults with autism spectrum disorder: A systematic review. Autism 28: 276-300. [Ref.]
- Elliott Jr RO, Dobbin AR, Rose GD, Soper HV (1994) Vigorous, aerobic exercise versus general motor training activities: effects on maladaptive and stereotypic behaviors of adults with both autism and mental retardation. J Autism Dev Disord 24: 565-576. [Ref.]
- Allison DB, Basile VC, MacDonald RB (1991) Brief report: comparative effects of antecedent exercise and lorazepam on the aggressive behavior of an autistic man. J Autism Dev Disord 21: 89-94. [Ref.]
- Morrison H, Roscoe EM, Atwell A (2011) An evaluation of antecedent exercise on behavior maintained by automatic reinforcement using a three-component multiple schedule. J Appl Behav Anal 44: 523- 541. [Ref.]
- Dishman RK, O’Connor PJ (2009) Lessons in exercise neurobiology: The case of endorphins. Ment Health Phys Act 2: 4-9.
- Portugal EMM, Cevada T, Monteiro-Junior RS, Guimarães TT, Rubini EC, et al. (2013) Neuroscience of exercise: from neurobiology mechanisms to mental health. Neuropsychobiology 68: 1-14. [Ref.]
- Meeusen R, Piacentini MF, Meirleir KD (2001) Brain microdialysis in exercise research. Sports Med 31: 965-983. [Ref.]
- Dietrich A, McDaniel WF (2004) Endocannabinoids and exercise. Br J Sports Med 38: 536-541. [Ref.]
- Iqbal M, Xiao XL, Zafar S, Yang PB, Si KW, et al. (2019) Forced Physical Training Increases Neuronal Proliferation and Maturation with Their Integration into Normal Circuits in Pilocarpine Induced Status Epilepticus Mice. Neurochem Res 44: 2590-2605. [Ref.]
- Bicker F, Nardi L, Maier J, Vasic V, Schmeisser MJ (2021) Criss-crossing autism spectrum disorder and adult neurogenesis. J Neurochem 159: 452-478. [Ref.]
- Saraulli D, Costanzi M, Mastrorilli V, Vecchioli SF (2017) The Long Run: Neuroprotective Effects of Physical Exercise on Adult Neurogenesis from Youth to Old Age. Curr Neuropharmacol 15: 519-533. [Ref.]
- Pęski MG, Gonçalves JT, Hébert JM (2021) Enriched Environment Promotes Adult Hippocampal Neurogenesis through FGFRs. J Neurosci 41: 2899-2910. [Ref.]
- Hendrikse J, Chye Y, Thompson S, Rogasch NC, Suo C, et al. (2022) Regular aerobic exercise is positively associated with hippocampal structure and function in young and middle-aged adults. Hippocampus 32: 137-152. [Ref.]
- Ledreux A, Håkansson K, Carlsson R, Kidane M, Columbo L, et al. (2019) Differential Effects of Physical Exercise, Cognitive Training, and Mindfulness Practice on Serum BDNF Levels in Healthy Older Adults: A Randomized Controlled Intervention Study. J Alzheimers Dis 71: 1245-1261. [Ref.]
- Voss MW, Prakash RS, Erickson KI, Basak C, Chaddock L, et al. (2010) Plasticity of brain networks in a randomized intervention trial of exercise training in older adults. Front Aging Neurosci 2: 32.
- Voss MW, Erickson KI, Prakash RS, Chaddock L, Kim JS, et al. (2013) Neurobiological markers of exercise-related brain plasticity in older adults. Brain Behav Immun 28: 90-99.
- Kronman CA, Kern KL, Nauer RK, Dunne MF, Storer TW, et al. (2020) Cardiorespiratory fitness predicts effective connectivity between the hippocampus and default mode network nodes in young adults. Hippocampus 30: 526-541. [Ref.]
- Rimmele U, Zellweger BC, Marti B, Seiler R, Mohiyeddini C, et al. (2007) Trained men show lower cortisol, heart rate and psychological responses to psychosocial stress compared with untrained men. Psychoneuroendocrinology 32: 627-635.
- Drogos LL, Edwards KW, Zhou R, Hall SE, Tyndall AV, et al. (2019) Aerobic exercise increases cortisol awakening response in older adults. Psychoneuroendocrinology 103: 241-248. [Ref.]
- Stranahan AM, Lee K, Mattson MP (2008) Central mechanisms of HPA axis regulation by voluntary exercise. Neuromolecular Med 10: 118-127. [Ref.]
- de Souza RF, de Moraes SR, Augusto RL, de Freitas Zanona A, Matos D, et al. (2019) Endurance training on rodent brain antioxidant capacity: A meta-analysis. Neurosci Res145: 1-9. [Ref.]
- Duman CH, Schlesinger L, Russell DS, Duman RS (2008) Voluntary exercise produces antidepressant and anxiolytic behavioral effects in mice. Brain Res1199: 148-158. [Ref.]
- Sun L, Sun Q, Qi J (2017) Adult hippocampal neurogenesis: An important target associated with antidepressant effects of exercise. Rev Neurosci 28: 693-703. [Ref.]
- Russo-Neustadt A, Beard RC, Cotman CW (1999) Exercise, antidepressant medications, and enhanced brain-derived neurotrophic factor expression. Neuropsychopharmacology 21: 679-682. [Ref.]
- Deslandes AC, Moraes H, Alves H, Pompeu FAMS, Silveira H, et al. (2010) Effect of aerobic training on EEG α asymmetry and depressive symptoms in the elderly: a 1-year follow-up study. Braz J Med Biol Res 43: 585-592. [Ref.]
- Song R, Grabowska W, Park M, Osypiuk K, Vergara-Diaz GP, et al. (2017) The impact of Tai Chi and Qigong mind-body exercises on motor and non-motor function and quality of life in Parkinson’s disease: A systematic review and meta-analysis. Parkinsonism Relat Disord41: 3-13. [Ref.]
- Hirsch MA, Toole T, Maitland CG, Rider RA (2003) The effects of balance training and high-intensity resistance training on persons with idiopathic Parkinson’s disease. Arch Phys Med Rehabil 84:1109- 1117. [Ref.]
- Bloomer RJ, Schilling BK, Karlage RE, Ledoux MS, Pfeiffer RF, et al. (2008) Effect of resistance training on blood oxidative stress in Parkinson disease. Med Sci Sports Exerc 40:1385-1389.
- Sutoo D, Akiyama K. (2003) Regulation of brain function by exercise. Neurobiol Dis 13: 1-14. [Ref.]
- Kemper TL, Bauman ML (1993) The contribution of neuropathologic studies to the understanding of autism. Neurol Clin 11:175-187. [Ref.]
- Kemper TL, Bauman ML (2002) Neuropathology of infantile autism. Mol Psychiatry 7: S12-13. [Ref.]
- Bailey A, Luthert P, Dean A, Harding B, Janota I, et al. (1998) A clinicopathological study of autism. Brain 121: 889-905. [Ref.]
- Aylward EH, Minshew NJ, Goldstein G, Honeycutt NA, Augustine AM, et al. (1999) MRI volumes of amygdala and hippocampus in non-mentally retarded autistic adolescents and adults. Neurology 53: 2145-2150. [Ref.]
- Abell F, Krams M, Ashburner J, Passingham R, Friston K, et al. (1999) The neuroanatomy of autism: a voxel-based whole brain analysis of structural scans. Neuroreport 10: 1647-1651. [Ref.]
- Tuchman R (2003) Autism. Neurol Clin N Am 21: 915-932.
- Martella G, Meringolo M, Trobiani L, De Jaco A, Pisani A, et al. (2018) The neurobiological bases of autism spectrum disorders: the R451Cneuroligin 3 mutation hampers the expression of long-term synaptic depression in the dorsal striatum. Eur J Neurosci 47: 701-708. [Ref.]
- Green SA, Hernandez L, Tottenham N, Krasileva K, Bookheimer SY, et al. (2015) Neurobiology of sensory overresponsivity in youth with autism spectrum disorders. JAMA Psychiatry 72: 778-786. [Ref.]
- Hazen EP, Stornelli JL, O’Rourke JA, Koesterer K, McDougle CJ (2014) Sensory symptoms in autism spectrum disorders. Harv Rev Psychiatry 22: 112-124. [Ref.]
- McCarty MJ, Brumback AC (2021) Rethinking stereotypies in autism. Semin Pediatr Neurol 38: 100897. [Ref.]
- Willsey AJ, State MW (2015) Autism spectrum disorders: from genes to neurobiology. Curr Opin Neurobiol 30: 92-99. [Ref.]
- Castelli F, Frith C, Happé F, Frith U (2002) Autism, Asperger syndrome and brain mechanisms for the attribution of mental states to animated shapes. Brain 125: 1839-1849. [Ref.]
- Thompson L, Thompson M, Reid A (2010) Functional neuroanatomy and the rationale for using EEG biofeedback for clients with Asperger’s syndrome. Appl Psychophysiol Biofeedback 35: 39-61. [Ref.]
- Hwang BJ, Mohamed MA, Brašić JR. (2017) Molecular imaging of autism spectrum disorder. Int Rev Psychiatry 29: 530-554. [Ref.]
- Segura M, Pedreño C, Obiols J, Taurines R, Pàmias M, et al. (2015) Neurotrophin blood-based gene expression and social cognition analysis in patients with autism spectrum disorder. Neurogenetics 16: 123-131. [Ref.]
- Taurines R, Segura M, Schecklmann M, Albantakis L, Grünblatt E, et al. (2014) Altered peripheral BDNF mRNA expression and BDNF protein concentrations in blood of children and adolescents with autism spectrum disorder. J Neural Transm 121: 1117-1128. [Ref.]
- Tostes MH, Teixeira HC, Gattaz WF, Brandão MA, Raposo NR (2012) Altered neurotrophin, neuropeptide, cytokines and nitric oxide levels in autism. Pharmacopsychiatry 45: 241-243. [Ref.]
- Bjørklund G, Meguid NA, El-Bana MA, Tinkov AA, Saad K, et al. (2020) Oxidative stress in autism spectrum disorder. Mol Neurobiol 57: 2314-2332. [Ref.]
- Chauhan A, Chauhan V, Brown WT, Cohen I (2004) Oxidative stress in autism: Increased lipid peroxidation and reduced serum levels of ceruloplasmin and transferrin - the antioxidant proteins. Life Sci 75: 2539-2549. [Ref.]
- Baker EK, Richdale AL, Hazi A, Prendergast LA (2019) Assessing a hyperarousal hypothesis of insomnia in adults with autism spectrum disorder. Autism Res 12: 897-910.
- Edmiston EK, Blain SD, Corbett BA (2017) Salivary cortisol and behavioral response to social evaluative threat in adolescents with autism spectrum disorder. Autism Res 10: 346-358. [Ref.]
- Corbett BA, Mendoza S, Wegelin JA, Carmean V, Levine S (2008) Variable cortisol circadian rhythms in children with autism and anticipatory stress. J Psychiatry Neurosci 33: 227-234. [Ref.]
- Kalueff AV, Stewart AM, Song C, Berridge KC, Graybiel AM, et al. (2016) Neurobiology of rodent self-grooming and its value for translational neuroscience. Nat Rev Neurosci 17: 45-59. [Ref.]
- Chmielewski WX, Beste C (2015) Action control processes in autism spectrum disorder--insights from a neurobiological and neuroanatomical perspective. Prog Neurobiol 124: 49-83. [Ref.]
- Peça J, Feliciano C, Ting JT, Wang W, Wells MF, et al. (2011) Shank3 mutant mice display autistic-like behaviours and striatal dysfunction. Nature 472: 437-442. [Ref.]
- Frackowiak J, Mazur-Kolecka B (2023) Intraneuronal accumulation of amyloid-β peptides as the pathomechanism linking autism and its co-morbidities: epilepsy and self-injurious behavior - the hypothesis. Front Mol Neurosci 16: 1160967. [Ref.]
- Muehlmann AM, Lewis MH (2012) Abnormal repetitive behaviours: shared phenomenology and pathophysiology. J Intellect Disabil Res 56: 427-440. [Ref.]
- Yoon SY, Kwon SG, Kim YH, Yeo JH, Ko HG, et al. (2017) A critical role of spinal Shank2 proteins in NMDA-induced pain hypersensitivity. Mol Pain 13: 1744806916688902. [Ref.]
- Farmer AL, Lewis MH (2023) Reduction of restricted repetitive behavior by environmental enrichment: Potential neurobiological mechanisms. Neurosci Biobehav Rev 152: 105291. [Ref.]
- Bortolato M, Floris G, Shih JC (2018) From aggression to autism: new perspectives on the behavioral sequelae of monoamine oxidase deficiency. J Neural Transm 125: 1589-1599. [Ref.]
- Wu Y, Meng YJ, Shi YF, Li JM, Xu YY, et al. (2023) Stereotactic neurosurgery as a symptomatic treatment for autism spectrum disorders: A systematic review. Asian J Psychiatr 83: 103541. [Ref.]
- Gouveia FV, Germann J, Devenyi GA, Morais RMCB, Santos APM, et al. (2020) Refractoriness of aggressive behaviour to pharmacological treatment: cortical thickness analysis in autism spectrum disorder. BJPsych Open 6: e85. [Ref.]
- Rajamani KT, Wagner S, Grinevich V, Harony-Nicolas H (2018) Oxytocin as a modulator of synaptic plasticity: Implications for neurodevelopmental disorders. Front Synaptic Neurosci 10: 17. [Ref.]
- Burrows EL, Laskaris L, Koyama L, Churilov L, Bornstein JC, et al. (2015) A neuroligin-3 mutation implicated in autism causes abnormal aggression and increases repetitive behavior in mice. Mol Autism 6: 62. [Ref.]
- Strekalova T, Svirin E, Veniaminova E, Kopeikina E, Veremeyko T, et al. (2021) ASD-like behaviors, a dysregulated inflammatory response and decreased expression of PLP1 characterize mice deficient for sialyltransferase ST3GAL5. Brain Behav Immun Health 16: 100306. [Ref.]
- Armstrong EC, Caruso A, Servadio M, Andreae LC, Trezza V, et al. (2020) Assessing the developmental trajectory of mouse models of neurodevelopmental disorders: Social and communication deficits in mice with Neurexin 1α deletion. Genes Brain Behav 19: e12630. [Ref.]
- Brodkin ES (2007) BALB/c mice: low sociability and other phenotypes that may be relevant to autism. Behav Brain Res 176: 53-65. [Ref.]
- Fazel Darbandi S, Robinson Schwartz SE, Qi Q, Catta-Preta R, Pai EL, et al. (2018) Neonatal Tbr1 dosage controls cortical layer 6 connectivity. Neuron 100: 831-845. [Ref.]
- Markopoulos A, Inserra A, De Gregorio D, Gobbi G (2022) Evaluating the potential use of serotonergic psychedelics in autism spectrum disorder. Front Pharmacol 12: 749068. [Ref.]
- Rojas-Charry L, Nardi L, Methner A, Schmeisser MJ (2021) Abnormalities of synaptic mitochondria in autism spectrum disorder and related neurodevelopmental disorders. J Mol Med 99: 161-178. [Ref.]
- Sterne JA, Savović J, Page MJ, Elbers RG, Blencowe NS, et al. (2019) RoB 2: a revised tool for assessing risk of bias in randomised trials. BMJ 366: l4898. [Ref.]
- Sterne JA, Hernán MA, Reeves BC, Savović J, Berkman ND, et al. (2016) ROBINS-I: a tool for assessing risk of bias in non-randomised studies of interventions. BMJ 355: 4919. [Ref.]
- Reichow B, Barton EE, Maggin DM (2018) Development and applications of the single-case design risk of bias tool for evaluating single-case design research study reports. Res Dev Disabil 79: 53-64. [Ref.]
- Goodman WK, Price LH, Rasmussen SA, Mazure C, Fleischmann RL, et al. (1989) The Yale-Brown Obsessive Compulsive Scale. I. Development, use, and reliability. Arch Gen Psychiatry 46: 1006- 1011. [Ref.]
- Silver JM, Yudofsky SC (1991) The Overt Aggression Scale: overview and guiding principles. J Neuropsychiatry Clin Neurosci 3: S22-S29. [Ref.]
- Rogers J, Renoir T, Hannan AJ (2019) Gene-environment interactions informing therapeutic approaches to cognitive and affective disorders. Neuropharmacology 145: 37-48. [Ref.]
- Hollander E, Soorya L, Chaplin W, Anagnostou E, Taylor BP, et al. (2012) A double-blind placebo-controlled trial of fluoxetine for repetitive behaviors and global severity in adult autism spectrum disorders. Am J Psychiatry 169: 292-299. [Ref.]
- McDougle CJ, Naylor ST, Cohen DJ, Volkmar FR, Heninger GR, et al. (1996) A double-blind, placebo controlled study of fluvoxamine in adults with autistic disorder. Arch Gen Psychiatry 53: 1001-1008. [Ref.]
- McDougle CJ, Holmes JP, Carlson DC, Pelton GH, Cohen DJ, et al. (1998) A double-blind, placebo-controlled study of risperidone in adults with autistic disorder and other pervasive developmental disorders. Arch Gen Psychiatry 55: 633-641. [Ref.]
- Dieleman GC, Huizink AC, Tulen JH, Utens EM, Creemers HE, et al. (2015) Alterations in HPA-axis and autonomic nervous system functioning in childhood anxiety disorders point to a chronic stress hypothesis. Psychoneuroendocrinology 51: 135-150. [Ref.]
- Dutcher JCD, Freeman SZ, Stein AT, Dinh M, et al. (2019) The effects of exercise on transdiagnostic treatment targets: A meta-analytic review. Behav Res Ther 115: 19-37. [Ref.]
- Boutcher SH, Landers DM. (1998) The effects of vigorous exercise on anxiety, heart rate, and alpha activity of runners and nonrunners. Psychophysiology 25: 696-702. [Ref.]
- Morres ID, Hatzigeorgiadis A, Stathi A, Comoutos N, Arpin Cribbie C, et al. (2018) Aerobic exercise for adult patients with major depressive disorder in mental health services: A systematic review and meta analysis. Depress Anxiety 36: 39-53. [Ref.]
- Hollocks MJ, Lerh JW, Magiati I, Meiser-Stedman R, Brugha TS, et al. (2018) Anxiety and depression in adults with autism spectrum disorder: A systematic review and meta-analysis. Psychol Med49: 559-572. [Ref.]
- APA (1987) Diagnostic and statistical manual of mental disorders. 3rd ed., Revised, American Psychiatric Association, Washington, DC, USA.
Download Provisional PDF Here
Article Type: RESEARCH ARTICLE
Citation: Im DS (2024) Exercise in the Treatment of Repetitive, Self-Injurious, and Aggressive Behavior in Adults with Autism Spectrum Disorder: A Neurobiological Analysis. J Neurol Neurobiol 9(1): dx.doi.org/10.16966/2379-7150.191
Copyright: ©2024 Im DS. This is an open-access article distributed under the terms of the Creative Commons Attribution License, which permits unrestricted use, distribution, and reproduction in any medium, provided the original author and source are credited.
Publication history:
SCI FORSCHEN JOURNALS
All Sci Forschen Journals are Open Access