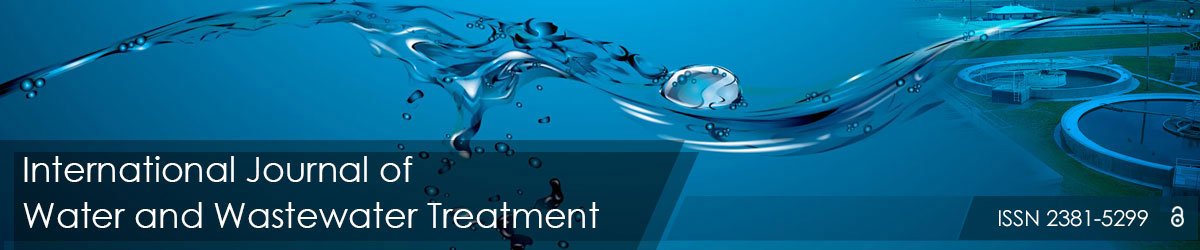
Full Text
Joachim Schneider Roger Babcock Jr* Tiow Ping Wong Bing Hu
Department of Civil and Environmental Engineering, University of Hawaiʻi at Mānoa, USA*Corresponding author: Roger Babcock Jr, Department of Civil and Environmental Engineering, University of Hawaiʻi at Mānoa, Honolulu, HI, USA, Tel: 808-956-7298; E-mail: rbabcock@hawaii.edu
Wastewater treatment plants are being transformed from energy sinks to energy-neutral or even energy-positive utilities. Conventional primary wastewater treatment diverts about half of the influent particulate organics to potential energy-generating anaerobic digestion but allows the soluble fraction to pass on to energy-consuming secondary oxidation. Enhanced removal of particulates can be accomplished via physical processes, but chemicals or biological processes are necessary for diversion of a portion of the soluble organics. The performance of biosorption (with waste activated sludge) followed by either flotation or micro screening separation has been evaluated in terms of some controllable operating parameters. Results show that the mixing ratio (or dose) of biosorbent, dissolved oxygen between 0.5 and 1.0 mg l-1 and contact times between 15 and 60 minutes have only minimal impact on soluble COD removal performance which can be from 30 to 35%. The use of polymer has a large impact on either DAF or micro screen separation in terms of TSS removal performance with increases from 30 to 50% without polymer to over 60% with polymer. Biosorption was found to follow zero-order kinetics for the truly soluble organics (ffCOD) fraction at a rate of 3.0 mg sorbed per minute per gram TSS sorbent which is mass transfer limited and does not reach equilibrium and thus cannot be fitted with an isotherm model. Sorption of the colloidal organic fraction (cCOD) is enmeshed in the floc matrix and is practically instantaneous.
Biosorption; Carbon diversion; Net-zero energy; Dissolved air flotation; Micro screens; Primary wastewater treatment; Kinetics; Polymer
During wastewater treatment, energy is both produced and consumed. In 2011, Wastewater Treatment Plants (WWTPs) accounted for 0.8 percent of the electricity consumption in the USA, half of which was used in secondary treatment for aeration [1]. Anaerobic digestion is commonly used to stabilize treatment sludges and can be a net producer of energy via capture, purification and combustion of methane in an engine-generator set. The goal of enhanced primary wastewater treatment is to divert the carbonenergy contained in the untreated influent from the aeration-bound liquids stream to the solids stream, where it can be harvested by means of anaerobic digestion. Two positive effects are thereby achieved: the oxygen demand and therefore electricity consumption in the secondary treatment is reduced, and the methane production and therefore energy scavenging in the anaerobic digester is increased. It is hypothesized that achieving net-zero energy for a WWTP is possible in this way [2] and it has been achieved in a plant in Austria [3].
Conventional primary wastewater treatment utilizing gravity sedimentation removes between 40 and 60 percent of Suspended Solids (TSS), and between 20 and 30 percent of Biochemical Oxygen Demand (BOD5) by capturing particulate organic matter but does not reduce the soluble fraction of raw influent organics. There is a current industry trend toward the use of screens and filter devices for enhanced capture/diversion of raw solids, which can achieve removals of up to 60-80 percent of particulate organics, but similarly do not reduce the soluble fraction [4]. Biosorption can be used to remove the soluble fraction. It is used in various industries for the removal of heavy metals [5] and organic pollutants [6], and is a fundamental cellular-level step in the activated sludge process prior to biochemical oxidation. Primary biosorption is a physico-chemical process in which Waste Activated Sludge (WAS), the sorbent, is mixed with Raw Wastewater (RW) in a small contactor where the particulate and soluble organic matters sorb onto/into the AS flocs/cells. The amount of sorption should be dependent on operational/physiological factors such as Dissolved Oxygen (DO) concentration, contact time, WASto-RW ratio, floc structure, sludge age/type, and/or the amount/ characteristics of Extracellular Polymeric Substances (EPS).
Research has found that the colloidal fraction of organic matter is targeted significantly more during biosorption than the particulate and truly soluble fractions [7]. It is assumed that this is because soluble matter diffuses into the cells while colloidal matter sticks to the floc matrix [8]. It was found that only aerobic sludge is suitable for biosorption, whereas neither primary nor digested sludge yielded positive results as sorbents [9].
Since the biosorption contactor effluent is higher in TSS than RW, an effective liquid-solid separation process is required to harvest the biosorbed materials. Potential separation processes include cloth filters, micro screens, and Dissolved Air Flotation (DAF), the latter two processes were compared here. The historical addition of nitrified WAS to primary clarifiers for co-thickening resulted in undesirable floating sludge, however, this makes DAF a good fit. The combination of biosorption with DAF has been evaluated in a few pilot-tests and at one full-scale facility, and was found to be capable of high removals of soluble BOD (20 to 30 percent) and TSS (more than 65 percent) while generating thick sludge (4 to 6 percent) in the DAF [10]. The use of micro screens as the separation step for biosorption has not been previously reported in the literature.
This study conducted bench-scale evaluations of biosorption for effects of contact time, DO, and WAS-to-RW mixing ratio on organic removal efficiency and also looked at sorption kinetics and potential isotherm relationships.
WAS and coarsely screened RW samples were collected no more than two hours before use and stored at ambient temperature during transportation to the lab (not chilled). Samples were taken at a regional WWTP receiving municipal influent with no industrial component which is designed to treat 13 Million Gallons per Day (MGD) by means of a Trickling Filter/Solids Contact process (TF/SC).
To simulate the biosorption process on a bench scale, RW and WAS were combined in a five-liter Plexiglass tank (contactor) and stirred at room temperature for 10 to 90 minutes on a stir plate, while DO concentrations were held constant at either 0.5 or 1.0 mg l-1 via diffused aeration control, and measured using Standard Method 4500-O G [11]. RW, WAS, and biosorption contactor effluent were characterized via Total Chemical Oxygen Demand (tCOD), Soluble COD (sCOD), Flocculated and Filtered COD (ffCOD) and Total Suspended Solids (TSS). Experiments were conducted with mixing ratios of five (MX5) or ten (MX10) percent WAS by volume, yielding doses of approximately 1.2 and 2.5 mg of WAS TSS per mg of RW sCOD, respectively. MX5 is a similar ratio of WAS TSS to RW sCOD that would occur at the subject TF/SC facility if all of the WAS and RW were combined in a biosorption contactor (ratio 1.16 to 1.34). Normalized removal was calculated similarly to Jorand F, et al. [7] by dividing the removed COD by the TSS added (see Equation 1).
Colloidal COD (cCOD) was defined as the difference between sCOD and ffCOD (see Equation 2).
In order to find the kinetics governing the biosorption process, replicate experiments were conducted for both 60- and 90-minutescontact time with sampling every ten minutes. These experiments utilized MX5 and 1.0 mgl-1 DO concentration. An attempt was made to find an isotherm relationship by running the biosorption experiment five times (with the same RW and WAS), each time using five liters of RW mixed with either 100, 200, 300, 400 or 500 ml of WAS. Experimental parameters for the isotherm experiment were 30 minutes of contact time and 1.0 mg l-1 DO concentration.
To simulate DAF separation, tap water was pressurized to 414 kPa (60 psi) and the pressurized vessel was vigorously shaken. 150 ml pressurized tap water was added to 850 ml of biosorption effluent in a one-liter graduated cylinder in which a float formed separately from the subnatant. After 3 minutes, the subnatant was sampled for COD and TSS and designated Lab DAF subnatant. Since DAF processes use pressurized DAF subnatant instead of tap water, the DAF separation was conducted a second time using pressurized Lab DAF subnatant instead of tap water and designated as DAF. For some experiments, CEP 414 cationic polymer was added and these are designated as PDAF. DAF was also conducted on RW samples alone.
A second solid-liquid separation method (micro screens) was evaluated by filtering biosorption effluent through wire mesh screens with openings of either 200 or 300 μm and sampling the filtrate. In some cases, 10 mgl-1 of CEP 414 cationic polymer was added before the filtration process, indicated by the letters PS before the screen size, while experiments without added polymer were labeled with the letter S. Micro screening was also conducted on RW samples alone.
COD was measured using Hach method 8000 which is based on Standard Method 5220D [10]. sCOD was measured by filtering the sample through a TSS glass fiber filter (pore size 1.5 μm) and measuring the COD of the filtrate. ffCOD was measured according to the method of Mamais D, et al. [12] using 30 ml of sCOD filtrate and 0.3 ml of zinc sulfate solution. TSS was measured using Standard Method 2540B [11]. PH was not measured in these experiments because it was found in prior pilot-scale tests to be unchanged due to the biosorption process. Statistical analyses including t-test, r2 and ANOVA were conducted in Microsoft Excel using the Data Analysis ToolPak Add-in.
Biosorption
Averages (± standard deviation) of biosorption contactor influent and effluent concentrations, removal percentages and normalized removal values for 53 experiments conducted with a 30-min contact time and DO of either 0.5 or 1.0 mg l-1 are shown in table 1. The data show that average truly soluble ffCOD removal is 21% while colloidal cCOD removal is much more efficient at 58%, however, this occurs because the amount of cCOD is much lower and the normalized removal values show that approximately the same net mass of cCOD and ffCOD are biosorbed per gram of adsorbent in 30 minutes contact time (90 mg sorbed gTSS-1).
Average Influent (RW) Concentration [mgl-1] | Average Effluent Concentration [mg l-1] | Average Removal [%] | Average Normalized Removal [mggTSS-1] |
|
sCOD | 150 ± 21 | 103 ± 18 | 31 | 178 ± 83 |
ffCOD | 113 ± 19 | 88 ± 18 | 21 | 89 ± 43 |
cCOD | 37 ± 12 | 15 ± 11 | 58 | 90 ± 83 |
Table 1: Average Biosorption Results for all 30-min Contact Time Experiments, n=53.
DO concentration effects
The effect of DO on biosorption was evaluated by testing two DO concentrations at 30 minutes of contact time. Average (± standard deviation) biosorption removal efficiencies at 0.5 and 1.0 mg l-1 DO are shown in table 2. The data indicate that within this range of DO values, the net mass of ffCOD biosorbed in 30 minutes contact appears to be larger when the DO is higher (93 versus 79 mg sorbed gTSS-1), however, due to variance, this difference is not statistically significant at α=0.05. The results suggest that DO concentrations in this range are not rate limiting. It is possible that lower DOs of perhaps 0.1 or lower would be rate limiting and adversely affect biosorption, however, such a determination was aim of the present study. Generally, providing greater DO than that resulting from the need for adequate mixing to provide contact between sorbent and adsorbate could facilitate undesirable bio-oxidation in the contactor.
Average Normalized Removal DO=0.5 [mggTSS-1] | Average Removal DO=0.5 [%] | Average Normalized Removal DO=1.0 [mggTSS-1] | Average Removal DO=1.0 [%] | P(T<=t) two-tail at α=0.05 | |
sCOD | 173 ± 85 | 30 | 180 ± 78 | 31 | 0.81 |
ffCOD | 79 ± 36 | 20 | 93 ± 45 | 22 | 0.23 |
cCOD | 94 ± 81 | 62 | 88 ± 84 | 56 | 0.82 |
Table 2: Average Biosorption Results for Different DO Concentrations at 30-min Contact Time, n=53.
WAS mixing ratio effects
The effect of the mass of sorbent on biosorption (the dose) was evaluated by testing two WAS mixing ratios; 5% WAS by volume (MX5) and 10% (MX10) at 30 minutes contact time. The MX5 and MX10 are equivalent to sorbent doses of 1.17 and 2.48mgTSS mgsCOD-1, respectively. Average (± standard deviation) biosorption removal efficiencies at MX5 and MX10 are shown in table 3. The data indicate that a doubled sorbent dose only slightly improved overall biosorption efficiency (sCOD from 29 to 31%, and ffCOD from 19 to 22%) in 30 minutes contact time and that mass adsorbed per unit mass of sorbent decreases, however, these differences are not statistically significant at α=0.05. This phenomenon could be explained as equilibrium not being achieved in 30 minutes of contact time. In full-scale operation, the mixing ratio (sorbent dose) would not be a significant variable parameter, since all of the WAS would be directed to the contactor followed by a separation/thickening step. While the contact time is a design variable, a contactor typically will not be sized for longer than 30 minutes of contact time. The experimental results show that it is not significantly beneficial to operate the overall system so as to generate larger sorbent doses.
Average Normalized Removal MX5 [mggTSS-1] | Average Removal MX5 [%] | Average Normalized Removal MX10 [mggTSS-1] | Average Removal MX10 [%] | P(T<= t) two-tail at α=0.05 | |
sCOD | 205 ± 88 | 29 | 165 ± 78 | 31 | 0.11 |
ffCOD | 96 ± 44 | 19 | 86 ± 42 | 22 | 0.45 |
cCOD | 110 ± 95 | 63 | 81 ± 76 | 55 | 0.28 |
Table 3: Average Biosorption Results for Different AS Mixing Ratios, n=53.
Biosorption kinetics
Zero-order kinetic coefficients were found for sCOD and ffCOD biosorption removals between 10 and 90 minutes of contact time (Figures 1 and 2), with 3.1 mg of sCOD and 3.0 mg of ffCOD removed on average per minute and per gram of TSS added (Table 4). The quality of the linear fits was indicated by r2 values of 0.7528 for sCOD and 0.6892 for ffCOD, and ANOVA Significance F values of less than 0.0104 for sCOD and 0.0191 for ffCOD (Table 4). No statistically significant fit was found for cCOD (Figure 3). Given that sCOD and ffCOD removal occur at very similar rates, and that cCOD removal seems to be unaffected by time, it is apparent that cCOD sorption occurs almost instantaneously upon the mixing of RW and WAS, while ffCOD sorption is a function of time. Guellil A, et al. [8] also found that cCOD removal reaches equilibrium prior to ffCOD removal [8]. It is possible that zero-order kinetics do not apply between zero and ten minutes of contact time, but this was not evaluated here because it is not thought to be practical to use less than 10 minutes contact time at full-scale. These results indicate that equilibrium is not achieved even at contact times of 90 minutes which is much greater than practical contact times of 15 to 30 minutes.
Figure 1: Kinetics of sCOD Removal.
Figure 2: Kinetics of ffCOD Removal.
Figure 3: Kinetics of cCOD Removal.
sCOD Kinetic Coefficient [mggTSS-1 min-1] | sCOD r2 | sCOD ANOVA Significance F | ffCOD Kinetic Coefficient [mggTSS-1 min-1] | ffCOD r2 | ffCOD ANOVA Significance F | |
1 | 3.7 | 0.8381 | 0.0104 | 3.5 | 0.7832 | 0.0191 |
2 | 2.4 | 0.9524 | 0.0009 | 2.7 | 0.8089 | 0.0147 |
3 | 2.4 | 0.7920 | 0.0013 | 1.6 | 0.6892 | 0.0056 |
4 | 4.0 | 0.7528 | 0.0024 | 4.1 | 0.8595 | 0.0003 |
Regr. | 3.1 | N/A | N/A | 3.0 | N/A | N/A |
Table 4: Kinetic Coefficients and Regression Information.
Isotherm
As WAS doses were increased from 2% to 10% (MX10), with a 30 minute contact time, a decrease of normalized removal was observed (see Figure 4). It is suggested that mass transfer limitations are the cause of this phenomenon. When the biosorption effluent (subnatant) was plotted as Ce versus the normalized biosorbed mass as qe, the observed relationship did not make the creation of a Freundlich isotherm possible. This is because equilibrium was not achieved within the given contact time which agrees with the kinetic experiments. No literature was found that was able to fit an isotherm to this type of biosorption, in agreement with our findings here [8].
Figure 4: Effects of WAS Dosage on sCOD, ffCOD, and cCOD Removal at 30-min Contact Time.
Separation
The biosorption contact process step was conducted prior to each separation process, and all removals mentioned here are in comparison to RW as shown in Equations 3 and 4.
When using screens without polymer, little to no tCOD and TSS removal was achieved (Table 5). Micro screens with openings of 300 μm (S300) removed zero percent tCOD and TSS, while 200 μm (S200) removed six percent of tCOD and zero percent TSS (Table 5). In fact, the amount of TSS and tCOD in the filtrate was larger than the RW because the added WAS sorbent contains a lot of TSS including the colloidal fraction. DAF averaged 49 percent tCOD and 34 percent TSS removal which is much better than the micro screens, but possibly less than a conventional primary clarifier. Addition of polymer significantly improved capture efficiency for both screens and DAF. Micro screens with openings of 200 μm in combination with polymer (PS200) removed 61 percent of tCOD and 55 percent of TSS on average while PS300 averaged 61 percent tCOD and 51 percent TSS removal. DAF with polymer, PDAF, achieved the highest average removals of 66 percent tCOD and 66 percent TSS. The different liquidsolid separation processes were found to yield similar sCOD removal rates, because the removal of sCOD occurs in the biosorption step and the separation step does not affect sCOD removal. Comparing PS300, which is thought to be a practical primary micro screen, with PDAF, indicates that the micro screens have only 5% lower sCOD removal, 4% lower ffCOD removal, and lower yet still likely acceptable TSS removal (51% versus 61%, see Table 5).
tCOD Removal Percentage [%] | sCOD Removal Percent [%] |
ffCOD Removal Percent [%] |
cCOD Removal Percent [%] |
TSS Removal Percentage [%] |
|
S200 | 14 | 32 | 23 | 50 | 0* |
S300 | 0* | 33 | 22 | 58 | 0* |
PS200 | 61 | 35 | 18 | 75 | 55 |
PS300 | 61 | 34 | 20 | 63 | 51 |
DAF | 49 | 29 | 25 | 31 | 34 |
PDAF | 66 | 39 | 24 | 68 | 66 |
Table 5: Average Removal Percentages of Biosorption with Different Separation Methods.
*tCOD and TSS in effluent was greater than influent due to the addition of WAS
Is Biosorption worth it?
In order to gauge the value of biosorption, results of biosorption experiments were compared to results of primary treatment processes that do not include a biosorption step, namely micro screens with and without polymer, and DAF without polymer. These methods are labeled here with WW in table 6. It was found that micro screens without polymer were only able to reduce tCOD by 30 to 32 percent and TSS by 42 to 48 percent, with no substantial effect on soluble organics. Micro screens with polymer reduced tCOD by 54 to 57 percent and TSS by 63 to 65 percent, including sCOD removals of 19 or 20 percent due to high cCOD removals, but no ffCOD removal. DAF achieved 60 percent tCOD and 63 percent TSS removal, also removing 11 percent of sCOD and 10 percent of ffCOD (Table 6).
tCOD Removal Percentage [%] |
sCOD Removal Percentage [%] |
ffCOD Removal Percentage [%] | cCOD Removal Percentage [%] |
TSS Removal Percentage [%] |
|
WW S200 | 32 | 1 | 4 | 0 | 48 |
WW S300 | 30 | 3 | 2 | 0 | 42 |
WW PS200 | 57 | 20 | 0 | 67 | 65 |
WW PS300 | 54 | 19 | 0 | 71 | 63 |
WW DAF | 60 | 11 | 10 | 17 | 63 |
Table 6: Average Removal Percentages without Biosorption.
Micro screens with openings smaller than 300 µm are likely unfeasible for use on RW due to fouling effects, therefore only S300 and PS300 are considered in figure 5. When comparing the results of the enhanced primary treatment methods evaluated here to the performance of a primary clarifier (PC), several observations can be made. First, biosorption with micro screen separation S300 is not feasible because TSS is not removed at all. Second, the addition of polymer (PS300) shows good removal of tCOD and TSS and some removal of sCOD. Third, DAF alone performs very similarly to PS300. Fourth, addition of biosorption to DAF produces much enhanced removal of soluble fractions but less TSS removal than a typical PC. Fifth, addition of polymer to DAF (PDAF) following biosorption provides about the same soluble fraction removal plus excellent TSS removal compared to DAF. Sixth, the combination of biosorption plus PS300 provides results that are comparable to biosorption plus PDAF, indicating that when using polymer, either micro screens or DAF could be used as the biosorption separation step.
Figure 5: TSS Removal Efficiencies of Different Liquid-Solid Separation Methods.
Adding WAS to RW to facilitate enhanced primary treatment via biosorption and carbon diversion might seem counterintuitive, however, it works. Using biosorption in combination with DAF and polymer, tCOD and TSS removals of 66 percent, along with about 30% sCOD removal (including about 20% of the truly soluble fraction, ffCOD), can be achieved. Also, using a micro screen (300 μm) with polymer as the separation step after biosorption gives the same removal of solubles and only slightly less but likely acceptable tCOD and TSS removals. It is noted that similar sCOD and TSS removal percentages are reported when using cloth media primary filtration [4] without a separate biosorption contactor, which means that the extra effort of adding WAS may not provide additional carbon diversion. However, there may be concerns regarding fouling and maintenance of primary cloth filters that do not exist for DAF and micro screens. It has also been shown here that biosorption removes a significant portion of ffCOD, whereas methods without biosorption do not. It is noted that the addition of polymer has a significantly positive effect on capturing TSS and all forms of COD in each process evaluated in this study
This research received funding from R.M. Towill Corporation.
- Kinyua MN, Elliott M, Wett B, Murthy S, Chandran K, et al. (2017) The role of extracellular polymeric substances on carbon capture in a high rate activated sludge A-stage system. Chem Eng 322: 428-434. [Ref.]
- Ding HB, Doyle M, Erdogan A, Wikramanayake R, Gallagher P (2015) Innovative Use of Dissolved Air Flotation with Biosorption as Primary Treatment to Approach Energy Neutrality in WWTPs. Water Pract Technol 10: 133-142. [Ref.]
- Crawford GV (2010) Best Practices for Sustainable Wastewater Treatment: Initial Case Study Incorporating European Experience and Evaluation Tool Concept. IWA Publishing 74. [Ref.]
- Caliskaner O, Techobanoglous G, Davis B, Rios J, Hazard J, et al. (2019) Evaluation of Biofiltration as an Advanced Primary Treatment Method. Proceedings of the Water Environment Federation WEFTEC. [Ref.]
- Comte S, Guibaud G, Baudu M (2006) Biosorption properties of extracellular polymeric substances (EPS) resulting from activated sludge according to their type: Soluble or bound. Process Biochem 41: 815-823. [Ref.]
- Wei D, Wang B, Ngo HH, Guo W, Han F, et al. (2015) Role of extracellular polymeric substances in biosorption of dye wastewater using aerobic granular sludge. Bioresour Technol 185: 14-20. [Ref.]
- Jorand F, Palmgren R, Block JC, Nielsen PH, Urbain V, et al. (1995) Biosorption of wastewater organics by activated sludges. Recent Progres en Genie des Procedes 44: 61-67. [Ref.]
- Guellil A, Thomas F, Block JC, Bersillon JL, Ginestet P (2001) Transfer of Organic Matter between Wastewater and Activated Sludge Flocs. Water Res 35: 143-150. [Ref.]
- Modin O, Saheb Alam S, Persson F, Wilen BM (2015) Sorption and release of organics by primary, anaerobic, and aerobic activated sludge mixed with raw municipal wastewater. PLoS One 10: e0119371. [Ref.]
- Doyle M, Erdogan A, Antonneau N, Johnson B, Babcock R, et al. (2018) Biologically enhanced primary treatment: A summary of experience from pilot, demonstration, and full-scale systems. Proceedings of the Water Environment Federation 2018: 496-522. [Ref.]
- Clesceri LS, Greenberg AE, Eaton AD (1999) Standard Methods for the Examination of Water and Wastewater. 20th Edition, APHA. [Ref.]
- Mamais D, Jenkins D, Pitt P (1993) A rapid physical-chemical method for the determination of readily biodegradable soluble COD in municipal wastewater. Water Res 27: 195-197. [Ref.]
Download Provisional PDF Here
Article Type: RESEARCH ARTICLE
Citation: Schneider J, Babcock Jr R, Wong TP, Hu B (2020) Effects of Mixing Ratio, Contact Time and Dissolved Oxygen on Efficiency of Biosorption for Primary Carbon Diversion. Int J Water Wastewater Treat 6(2): dx.doi.org/10.16966/2381-5299.167
Copyright: © 2020 Babcock Jr R, et al. This is an open-access article distributed under the terms of the Creative Commons Attribution License, which permits unrestricted use, distribution, and reproduction in any medium, provided the original author and source are credited.
Publication history:
SCI FORSCHEN JOURNALS
All Sci Forschen Journals are Open Access