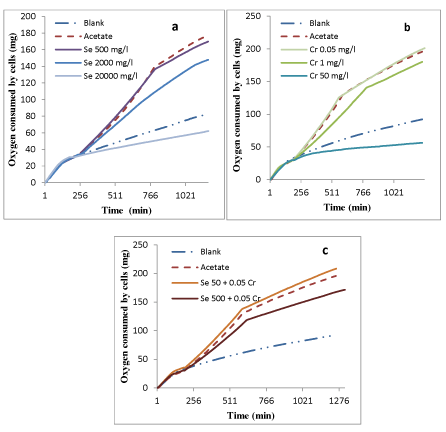
Figure 1: Respiration profiles of activated sludge exposed to a) Se b) Cr6+ and c) combination of 3 Se/ Cr6+.
Sepideh Sadeghi* Suzette Burckhard Christopher Schmit Beverly Klein
Department of Civil and Environmental Engineering, South Dakota State University, USA*Corresponding author: Sepideh Sadeghi, Department of Civil and Environmental Engineering, South Dakota State University, USA, E-mail: sepideh.sadeghi@sdstate.edu
The toxic impact of combined selenium (Se) and hexavalent chromium (Cr6+) salts on activated sludge from a local wastewater treatment facility has been studied through respiration inhibition tests. Results indicated that Se had inhibitory effects at concentration values above 500 mg Se/L, while, Cr6+ inhibited acetate biodegradation above a concentration of 0.05 mg Cr6+/L. The effects of the combination of Se and Cr6+ on activated sludge was also found to be inhibitory for acetate biodegradation; 20% biomass respiration inhibition was achieved at the combination of 500 mg Se/L with 0.05 mg Cr6+/L. However, the biomass performance for acetate removal was enhanced by 10% using 50 mg Se/L combined with 0.05 mg Cr6+/L. The effect of heavy metals on microbial communities’ metabolic activity in activated sludge was also investigated using EcoPlatesTM. The results showed that the presence of Cr6+ increased the activated sludge acclimation time for metabolic activity compared to the presence of Se. The highest and the lowest functional diversity were recorded for activated sludge without added heavy metals and activated sludge fed with Cr6+, respectively.
Respirometry; Acetate biodegradation; Combination effect of heavy metals; EcoPlatesTM
Biological treatment methods are the basis of wastewater treatment worldwide. The control of the treatment process is complicated due to the large number of parameters that can affect it. Heavy metals in wastewater influent can be stimulatory, inhibitory, or even toxic in biochemical reactions depending on their concentrations [1-3]. Cu, Zn, Pb, Ni, and Co are required by some organisms in low amounts (µg/L) as cofactors for enzymatic activities. However, heavy metal ion concentrations at the level of mg/L are known to be toxic for most organisms [4]. Toxicity can be attributed to the chemical binding of heavy metals to enzymes and microorganisms [5], resulting in the disruption of enzyme structure and activities [6,7]. Variation in enzymatic activity can be indicative of the changes occurring in the bacterial communities [8]. Heavy metals can also form undefined compounds in relatively high concentrations which lead to cytotoxic effects [9] that can change the biochemistry and performance of the activated sludge for the decomposition of organic matter.
Heavy metals, such as Selenium, can be present in the natural environment. Soils containing high concentrations of selenium are commonly found in many parts of the world including North America, Western Canada, China, Sweden, Italy, Finland, and Ireland [10]. The Dashan Region of China has reported selenium concentrations in the range of 0.31 and 7.65 µg/kg (dry weight) [11]. Lévesque found selenium concentrations in the range of 0.073 to 2.090 mg/kg [12]. Selenium has been reported in relatively high concentrations in western South Dakota (USA) soils [10,13,14]. Selenium concentrations in South Dakota soils ranged from below detection limit to 12.6 mg/kg with an average of approximately 6 to 7 mg/kg at a depth of 25.4 cm [14], and an average of 7.5 mg/ kg in 0-5 cm of some South Dakota soils [10]. One consequence of higher selenium concentrations in native soils is their prevalence in water, especially groundwater [15], and runoff due to natural and anthropogenic activities. Selenium has been found in fish and fauna in areas affected by runoff from mining activities and oil and gas development [16].
Selenium is highly soluble in water with primary species; selenate (SeO42-), which is readily mobile and bioavailable for microorganisms, and selenite (SeO32-), which is less mobile and bioavailable [10]. Selenium can be transferred to surface water, groundwater, and sewage systems from irrigation and stormwater runoff [10,17,18]. Furthermore, high selenate concentrations from the stormwater runoff are readily bioavailable for microorganisms in sewage systems. Moreover, wastewater treatment systems often receive more than one metal simultaneously [19]. Hexavalent chromium is one of the toxic heavy metals which come from various industrial activities such as plating facilities, power plants, mining, electrical equipment manufacturing, leather and wood industry, surface treatment or production of fertilizers [20,21], and can subsequently be released to sewage systems which flow to municipal wastewater treatment plants. Concentrations of Cr6+ in wastewaters vary significantly (from 50 to 700 mg/L), depending on industrial activities, and were reported in the range of 0.033-5.25 mg/L in surface waters and groundwater of some industrial areas [22]. However, the concentrations of Cr6+ in wastewater are predicted to be considerably lower since industrial effluents would be diluted by domestic wastewaters.
A few studies addressed the toxic influence of heavy metals on activated sludge of municipal wastewater treatment plants [4,19,23- 25]. Tyupa DV, et al. [19] reported lethal doses of silver at 1, 50, 300, and 600 µg/L and lethal doses of uranium at 120, 1,500, and 1,000 mg/L, respectively, in activated sludge from a wastewater treatment plant. Liu G and Wang J [24] investigated the effect of nano-copper and nanomagnesium particles on activated sludge. Their results indicated that copper had half maximum inhibitory concentration values of 19 and 5.5 mg/L for endogenous respiration and BOD biodegradation. According to Vaiopoulou and Gikas [25] a number of reports supported that Cr6+ is toxic to activated sludge at concentrations above 5 mg/L, while others reported growth stimulation at concentrations up to 25 mg/L. However, all reports agreed that Cr6+ is definitely an activated sludge growth inhibitor at higher concentrations, with 80 mg Cr6+/L being identified as a lethal dose.
A number of methods have been suggested for measuring metal toxicity in microbial systems [26]. The most common method for aerobic microorganisms is the measurement of microbial respiration rate [25,27]. Respiration is an essential activity of aerobic bacteria and respirometry methods are used to determine wastewater toxicity to bacterial populations in activated sludge. Toxic compounds can cause a decrease in the respiration rate of activated sludge microorganisms in respirometric tests. Bacterial respiration rate is typically measured by the oxygen consumption rate in a respirometer [4]. Additionally, activated sludge microbial communities and metabolisms may be affected by the presence of heavy metals [28]. There are some methods for examination of mixed bacterial populations. Bacteria can be examined under microscopes, DNA techniques, culturing and chemical tests [29]. EcoPlatesTM are designed for microbial community analysis without specifically identifying particular bacterial species. EcoPlatesTM have 95 individual carbon sources plus a water control on a 96 well plate that allows rapid determination of the metabolic capabilities of a bacterial population without time-consuming reagent preparation. They are easy to use and provide beneficial information about bacterial communities exposed to toxic compounds in a relatively short time [8,29].
The fact that the extensive amount of selenium in soils results in elevated levels of selenium in surface waters due to natural and increasingly anthropogenic activities such as mining and oil and gas development, reveals a need to investigate the detrimental dosages of selenium on aerobic activated sludge bacteria. Moreover, since wastewater treatment systems often receive more than one metal simultaneously, the synergistic effects of selenium with another heavy metal found in the industrial wastewater, Cr6+ was also examined in this study. Additionally, the structure and function of bacterial population in the presence of heavy metals has rarely been documented in recent studies. Thus, the present work was to investigate the individual and combined effects of Se and Cr6+ on the microbial communities’ function and structure.
The biomass used for this research was collected from an activated sludge aeration basin of a local wastewater treatment facility. The facilities’ mixed liquor activated sludge averages are 2000 mg/L measured as chemical oxygen demand (COD) with a mixed liquor suspended solid (MLSS) of 1866 mg/L and a mixed liquor volatile suspended solid (MLVSS) of 1400 mg/L. Activated sludge characteristics are summarized in table 1. Four solutions; two mineral base solutions, a nutrient solution, and a buffer solution were prepared in the laboratory according to [30] to support the biomass growth in aerobic respirometric tests. These solutions ensure that the biomass is able to fully consume the substrate without lacking minerals. The buffer solution contained 30% (w/v) potassium hydroxide (KOH; 85.8% purity) obtained from Fisher Chemical and was applied to each bottle to remove CO2 from the headspace to ensure that 100% oxygen was being measured by the respirometer. Sodium acetate (C2 H3 O2 Na; 99% purity) obtained from Fisher Scientific (Fairlawn, NJ) was used as substrate for this study. Sodium selenate (Na2 O4 Se; 98% purity) obtained from Acros Organics and liquid hexavalent chromium (Cr6+) obtained from Ricca Chemical Company (Arlington, TX) were added to the tests as heavy metals. All material stock solutions were prepared by dissolving the required amount of respective salts into distilled water. EcoPlatesTM were obtained from BIOLOG Company (Hayward, CA) and were used to assess microbial community structure and response.
Sludge | ||
Liquid phase | Solid phase | |
pH | 8 | - |
DO (mg/L) | 6 | - |
Temperature | 16°C | - |
Cr6+ | NA | 34 mg/kg dry weight |
Se | 0.24 mg/L | 44 mg/kg dry weight |
COD | 2000 mg/L | - |
MLSS | 1866 mg/L | - |
MLVSS | 1400 mg/L | - |
Table 1: Activated sludge characterization
A PF-8000 pulse flow respirometer was used for this research. This apparatus is designed specifically to measure oxygen uptake for aerobic respirometry, and gas production for anaerobic, and anoxic biological reactions. The apparatus is an eight position PF-8000 respirometer. The respirometer was associated with a laptop computer which uses the respirometry software, a pressure regulator module (PR-5010 model), a respirometer control module, a water bath cooler, stirring base, incubator, vessels, syringe, and oxygen tank. A BioLog MicroStation microplate reader was used to measure EcoPlatesTM.
Activated Sludge (AS) was collected from the local WWTP aeration basin. The test was started within 50 minutes holding time, just after the sludge was brought to the laboratory in order to preserve the sludge characteristics. Upon returning to the laboratory all respirometry equipment was powered on. The water bath cooling unit was set to room temperature at 20 ± 0.1°C and turned on approximately 30 minutes prior to the start of the test to allow the water to stabilize to the prescribed temperature. Meanwhile, the activated sludge sample was bubbled with air for 30 minutes to remove any remaining soluble substrate. A dose of 2.13, 8.52, and 0.71 mL/L of each of the mineral bases I & II, buffer, and nutrient base, respectively, was added to each of the eight bottles based on the calculation used in [30]. The activated sludge sample was shaken well before pouring into the bottles in order to maintain a homogenous mixture. A volume of 500 mL of this sludge mixture was added to the 500 mL bottles by using a plastic cylinder and funnel. In each of the carbon-dioxide adsorption tubes, 5 mL of 30% (W/V) KOH was added and then the tube was placed into the bottle. Oxygen uptake rate was recorded every 1 minute. After a few hours, the oxygen uptake rate became constant (endogenous respiration was reached), 210 mg acetate (based on the calculation used in [31]) along with the different concentrations of heavy metals (0.05-50 mg/L Cr6+ and 500-20,000 mg/L Se) were dosed to each bottle. Higher concentrations of selenium were only used in this study to investigate the dose range of selenium which was toxic for activated sludge microorganisms, and was not necessarily used to mimic the level of selenium in a municipal sewage system. The biomass then utilized the substrate consuming oxygen. The oxygen uptake reached its maximum level when all the substrate was consumed by the biomass and then the endogenous decay respiration rate returned. In every set of tests, there was one blank to measure the background oxygen consumption from endogenous decay. After substrate utilization was finished and the oxygen usage rate returned to background levels, the test was stopped and data was analyzed. The supernatant from each bottle was collected to measure the selected heavy metal using ICP Optical Emission Spectrometer (Varian 720-ES, Australia).
The EcoPlatesTM test has 96-well cell culture plates that contained 31 important carbon sources repeated three times along with controls [29]. The wells were inoculated with freshly collected sludge from the batch test that had been centrifuged, rinsed, and resuspended in 0.85% (w/v) NaCl (using the method of [28]), and diluted 10 times. Plates were incubated at ambient temperature (20°C) and read by microplate reader between 24 and 72 hours following inoculation.
Each batch inhibition experiment was repeated at least three times in this study. Three main parameters; COD, MLSS, and MLVSS, of activated sludge were measured for each new sludge obtained according to the method 5220 C, 2540D, and 2540 E, respectively, by using Standard Methods for the Examination of Water and Wastewater [32]. Chromium and selenium residual were measured based on the EPA 200.7 and 3015 methods [33]. EcoPlatesTM data analysis is defined by [29].
Oxygen consumed by cells is indicative of biomass activity and pollutant biodegradation, and respirometers are devices that measure the oxygen consumed by living organisms for substrate biodegradation [30]. Selenium, Cr6+ and the combination of these were tested at different concentrations, determining their inhibitory and lethal effects on activated sludge performance to remove acetate. Figure 1 shows the inhibition profile observed in the respirometric tests for the two tested metals and their combination.
Figure 1: Respiration profiles of activated sludge exposed to a) Se b) Cr6+ and c) combination of 3 Se/ Cr6+.
Before sodium acetate stock solution was added to the process at ~180 minutes, all oxygen consumption curves almost overlapped as the organisms were in endogenous respiration. After the addition of sodium acetate, the oxygen consumption in the reactor fed only with acetate (Figure 1 a,b,c) appreciably increased as a result of acetate biodegradation. The rate of oxygen uptake was found to be 0.2 ± 0.02 mg O2/mg COD-h for acetate removal. No considerable inhibition was observed for 500 mg/L Se (Figure 1a), however, acetate degradation rate was inhibited by 2000 mg/L Se with an oxygen uptake rate of 0.13 ± 0.02 mg O2/h (35% respiration inhibition). The lethal impact of selenium on bacteria was determined by increasing the selenium concentration to 20,000 mg/L. This concentration led to bacterial mortality and a decrease in oxygen consumption that led to a rate that was less than that experienced by the blank test (endogenous respiration only). A reason for activated sludge resistance to elevated concentrations of Se can be defined by microbial reduction of selenium species in activated sludge. Two oxyanions:selenate (SeO42-) and selenite (SeO32-) are reduced to selenide (Se2-) which is less toxic than SeO42- or SeO32- to microbial cells. In the presence of excess available Se, cells begin also to incorporate Se instead of S into cellular components that normally contain S [34].
As depicted in figure 1b, acetate removal was not inhibited by 0.05 mg/L Cr6+, whereas, 1 mg/L Cr6+ inhibited acetate removal with the rate of 0.15 ± 0.02 mg O2/h (25% respiration inhibition), and as the Cr6+ concentration increased to 50 mg/L, the oxygen consumption decreased to the rate that was less than the blank test. According to the results, Cr6+ toxicity occurred at a lower concentration (50 mg/L) compared to Se (20,000 mg/L). Hartmann S, et al. [4] reported similar results as Cr6+ was one of the most toxic heavy metals for aerobic activated sludge with a 52 % respiration inhibition above a dose of 10 mg/L. Other studies on Cr6+ effects on activated sludge reported conflicting results. Some of them mentioned that activated sludge growth is inhibited at Cr6+ concentrations above 5 mg/L, while others report growth inhibition at concentrations above 25 mg/L. However, all researchers agree that Cr6+ is definitely an activated sludge growth inhibitor at higher concentrations [4,19,24,35].
Based on the results, Se and Cr6+ were not inhibitory for acetate removal at 500 mg/L and 0.05 mg/L, respectively. To determine whether the combination of non-inhibitory doses of Se and Cr6+ can adversely affect activated sludge performance in the biodegradation process, the bottles were dosed with 500 mg/L activated sludge and a lower concentration (50 mg/L) of Se combined with 0.05 mg/L Cr6+. Figure 1c, shows that 500 mg/L Se, combined with 0.05 mg/L Cr6+, inhibited biomass respiration with the rate of 0.16 ± 0.02 mg O2/h (20% respiration inhibition). This can be attributed to the combination effect of Se and Cr6+ on activated sludge performance for acetate biodegradation. However, the biomass performance for acetate removal was enhanced by 10% (the rate of 0.22 ± 0.02 mg O2/h) using 50 mg/L Se combined with 0.05 mg/L Cr+6. According to this result, Se stimulated biomass growth. The lower Se concentration (50 mg/L) in combination with 0.05 mg/L Cr+6 achieved higher biomass growth. Little was known about the impact of multiple metals on microorganisms. According to Chu K W and Chow KL [36] metals’ covalent and ionic indexes explain the interactions between metals. It appears metals with higher covalent index tend to have synergistic effect and metals with low covalent index tend to neutralize the toxic effect of other metals with lower covalent index. Se and Cr ionic and covalent indexes were not specified previously [37], hence would not allow confirmation with our experimental results, so further study is required to investigate the synergistic mechanism that exists between Se and Cr.
Other studies have shown synergistic effects of metals. Dilek FB, et al. [23] studied the combined effect of Ni and Cr6+ on activated sludge process in terms of COD removal. According to their results the combination of Ni and Cr6+, did slightly affect activated sludge performance in terms of COD removal efficiency. According to Dawson PS and Jenkins SH [38], the combination of Ni and Cu depressed oxidation in a respirometric study. Mn paired with Cd or Zn acted synergistically, however, no synergistic impacts were observed when Cyanide was paired with Cu or Ni, or Cu paired with Fe or Cr. Chu and Chow [36] reported synergistic effects in pairwise tests for Cr, Cd and Cu. They found that the mortality rate increased when metals were combined, and they argued that since the combination of a small amount of each metal could generate a severe lethal impact, the potential metal interaction in environmental samples should not be neglected. The combined effect of four metals was also studied by Barth EF, et al. [39]. They found that four metals (Cu, Cr, Ni and Zn) in a total concentration of 8.9 mg/L had no adverse effect on the overall efficiency of a pilot-scale activated sludge plant.
Microbial communities’ variation in activated sludge with the presence of heavy metals has been investigated using EcoPlatesTM. EcoPlatesTM generally follow the same color development patterns with incubation time but varied for different activated sludge samples [8]. Results from EcoPlatesTM are shown in figure 2 for 35 hours of incubation time. The activated sludge microbial community fed only with acetate, and fed with acetate and Se (2000 mg/L) developed faster compared to the activated sludge microbial community fed with acetate and Cr6+ (1 mg/L), and the metals combination of 500 mg/L Se and 0.05 mg/L Cr6+ as more wells showed color development when compared. Gryta A, et al. [8] reported similar results for heavy metal contaminated sludge. They found that waste activated sludge which was not contaminated by heavy metals had a stronger metabolic activity to utilize carbon substrates on EcoPlatesTM.
Figure 2: a)AS fed with acetate, b)AS fed with acetate & Se, c)AS fed with acetate & Cr, d)AS fed with acetate and combination of metals in 35 h. (Metal concentration: Se: 2000 mg/L, Cr6+:1 mg/L, Se/Cr6+: 500/0.05 mg/L).
Activated Sludge (AS) fed only with acetate, and fed with acetate and 2000 mg/L Se were found to have the highest metabolic activity, and were able to metabolize 25 and 24 out of 31 substrates, respectively, while AS fed with acetate and 1 mg/L Cr6+ only metabolized 18 out of 31 substrates and AS fed with acetate and the combined metals metabolized 22 out of 31 substrates. The community in the AS fed with only acetate was able to utilize the most substrates; however, there were six substrates that were utilized at a lower level than the majority of other substrates (i-Erythritol, L-Phenylalanine, 2-hydroxybenzoic acid, L-Threonine, Itaconic Acid, and α-Ketobutyric Acid). The lowest utilization of all carbon sources was recorded for AS fed with acetate and Cr6+, which was unable to utilize L-Arginine, D-Xylose, Pyruvic Acid Methyl Ester, i-Erythritol, 2-Hydroxy Benzoic Acid, L-Phenylalanine, α-Cyclodextrin, L-Threonine, D- Glucosaminic Acid, Itaconic Acid, α-Ketobutyric Acid, Phenylethylamine, and D,L-α -Glycerol Phosphate. These results are indicative of chromium impact on bacteria, which led to the deterioration in the microbial activity and diversity of substrate utilization and might be due to a reduction in catabolic functions [8]. The five substrates which none of the communities oxidized were i-Erythritol, 2-Hydroxy Benzoic Acid, L-Phenylalanine, Itaconic Acid, α -Ketobutyric Acid.
The total number of substrates used, percent functional diversity, and percent variation of results within samples for activated sludge fed only with acetate, acetate and Se, acetate and Cr6+, acetate with the combination of two metals are shown in table 2. Activated sludge without the inhibitory metals had the highest functional diversity which is indicative of high species diversity. The lowest functional diversity belonged to the activated sludge fed with Cr6+ due to its toxicity. Activated sludge without inhibitory metals and AS fed with Se did not have a large proportion of variation on EcoPlatesTM, however, AS fed with Cr6+ and fed with the combined metals had a large proportion of variation.
AS fed with acetate | AS fed with acetate and Se | AS fed with acetate and Cr6+ | AS fed with acetate and combination of metals | |
Total # of substrate used | 25 | 24 | 18 | 22 |
% Functional Diversity | 80.6% | 74.2% | 61.3% | 74.2% |
% Variation of Results within Sample | 3.2% | 6.45% | 35.5% | 22.6% |
Table 2: EcoPlatesTM calculations. (Metal concentration: Se: 2000 mg/L, Cr6+: 1 mg/L, Se/Cr6+: 500/0.05 mg/L).
Hexavalent chromium residual concentrations in the respirometer supernatant are reported in table 3. The Cr6+ residuals in the supernatant were reduced 89% (compared to blank) when the activated sludge was only fed with acetate. However, Cr6+ residual in the supernatant is increased from 0.013 to greater than 5 mg/L by increasing the initial Cr6+ concentration from 0.05 to 50 mg/L. The combination of Se and Cr6+ showed that as the initial Se concentration increased from 50 to 500 mg/L, the Cr6+ residual in the supernatant slightly decreased. It is possible that the increasing Se concentration may enhance chromium precipitation. However, further study is required to investigate the fate of metals in the sludge sediment. Hexavalent chromium removal in the activated sludge might be attributed to two potential mechanisms; chromium reduction and accumulation in activated sludge and adsorption on cells [40]. The mechanism associated with chromium removal is the reduction of Cr6+ to Cr3+. The Cr3+ is then precipitated as Cr(OH)3 and accumulated in the activated sludge [27,41]. Stasinakis AS, et al. [27] reported that the oxidation of Cr3+ to Cr6+ is so slow that Cr3+ may be adsorbed on solids before being oxidized [27]. The adsorption mechanism could be attributed to MLSS content as higher MLSS concentrations allowed for more adsorption sites to be available for metal uptake, and higher chromium removal can be achieved by adsorption on the extracellular polymers or the cytoplasm and cell wall [27,40].
Sample type | Cr6+ Residual (mg/L) |
Blank | 0.009 |
only acetate | 0.001 |
Cr6+ 0.05 mg/L | 0.013 |
Cr6+ 0.1 mg/L | 0.020 |
Cr6+ 1 mg/L | 0.035 |
Cr6+ 5 mg/L | 2.269 |
Cr6+ 10 mg/L | 4.746 |
Cr6+ 50 mg/L | >5 |
Se/Cr6+: 50/0.05 mg/L | 0.015 |
Se/Cr6+: 100/0.05 mg/L | 0.011 |
Se/Cr6+: 200/0.05 mg/L | 0.010 |
Se/Cr6+: 500/0.05 mg/L | 0.010 |
Table 3: Hexavalent chromium residual in the supernatant.
Extensive amounts of Se have been reported in soils in western South Dakota as well as China and Canada which presents the possibility that Se finds its way to surface water, groundwater, and even sewage systems. Moreover, because wastewater treatment plants often receive more than one metal simultaneously, this paper determined the toxic dosage of Se and Cr6+ individually and together on activated sludge bacteria population. The results showed that the most toxic heavy metal in this study was Cr6+. Hexavalent chromium was inhibitory (25%) for acetate biodegradation at a dose of 1 mg/L and was toxic at a dose of 50 mg/L. Selenium also inhibited acetate biodegradation with a 35% respiration inhibition at a concentration of 2000 mg/L. However, Se toxicity occurred at a considerably higher concentration of 20,000 mg/L. The combination of Se and Cr6+ showed respiration inhibition at concentrations of 500 mg/L and 0.05 mg/L, respectively.
The results of EcoPlatesTM showed that the highest metabolic activity was for AS fed only with acetate, and fed with acetate and Se, while Cr6+ led to deterioration in microbial activity and diversity of substrate. Future investigation is required to determine which bacteria are affected by the heavy metals toxicity. Additionally, according to the results it appeared that Se could enhance Cr6+ precipitation in activated sludge. Overall, the results of this study can provide beneficial information on wastewater treatment systems’ microorganisms in the presence of heavy metals for the areas of the world that have a high background level of heavy metals in the native soils.
This material is based upon work supported by the National Science Foundation (NSF) under Grant Number NSF 1642037. Any opinions, findings, and conclusions or recommendations expressed in this material are those of the authors and do not necessarily reflect the views of the NSF or of the colleges and universities with which the authors are affiliated. The authors also like to thank WEERC lab and Civil & Environmental Eng. Department of South Dakota State University.
Download Provisional PDF Here
Article Type: RESEARCH ARTICLE
Citation: Sadeghi S, Burckhard S, Schmit C, Klein B (2019) A Study of Toxic Dosage of Combined Selenium and Hexavalent Chromium on Activated Sludge Bacteria. Int J Water Wastewater Treat 5(1): dx.doi.org/10.16966/2381-5299.161
Copyright: © 2019 Sadeghi S, et al. This is an open-access article distributed under the terms of the Creative Commons Attribution License, which permits unrestricted use, distribution, and reproduction in any medium, provided the original author and source are credited.
Publication history:
All Sci Forschen Journals are Open Access