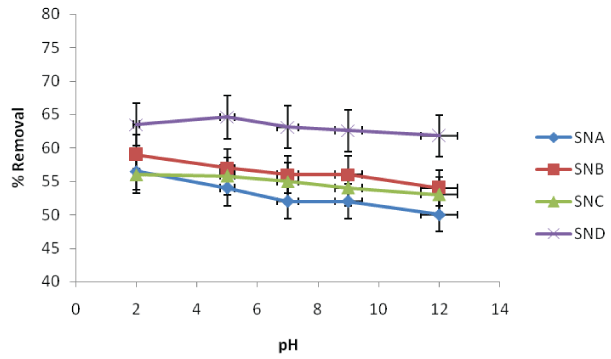
Figure 1: Effect of pH on the removal fluoride ions using SNA, SNB, SNC and SND
Adamu Abdulhameed* Harun M Mbuvi Evans O Changamu
Chemistry Department, Kenyatta University Nairobi, Kenya*Corresponding author: Adamu Abdulhameed, Chemistry Department, Kenyatta University Nairobi, Kenya, E-mail: adamhameed10@gmail.com
Adsorption of fluoride was done from its aqueous solution by using silicon nitride. In this study, silicon nitrides, SNA, SNB, SNC and SND were synthesized by hydrothermal process done at temperature of 100°C, 150°C, 200°C and 300°C using Rice husk ash (RHA) and sugarcane bagasse ash (SBA) respectively. A batch study on fluoride sorption was carried out at various experimental conditions, including different pH, initial fluoride concentration, and adsorbent dose, shaking speed, contact time and temperatures. Optimum adsorption efficiencies were achieved at pH 2 for SNA, SNB, SNC and pH 4 for SND, contact time 100 minutes, temperature 25°C and shaking speed 600 rpm. The sorption data was found to best into Langmuir isotherm. The adsorption capacities for fluoride ions were 7.9, 8.9, 13.9 and 49.8 mg/g for SNA, SNB, SNC and SND respectively. This results indicate that the silicon nitride constitute a promising material for the development of an adsorption technology for the removal of fluoride ions from aqueous systems.
Silicon nitride, Fluoride ion, Adsorption isotherm
Fluoride ion contamination of ground water has been recognized in numerous parts of world [1]. The common source of fluoride ions contaminations happens through soil and rock arrangement as fluorapatite, fluorspar and amphiboles, geochemical stores, normal water system and earth crust [2]. Wastewaters containing high measures of fluoride ions are generated by warm power plants; rubber, glass, fertilizer, ceramic productions; and electroplating industries (WHO, 1997).
Fluoride ion is viewed as helpful at optimum levels. It is vital for the strongness of bones and teeth. Fluoride expands tooth mineralization and diminishes dental veneer demineralization and dentine touchiness. On the other hand at elevated amounts it leads to mottling and embrittlement of teeth, a condition called dental fluorosis. Bones get to be denser, harder, and weaker. Extended exposure of fluoride ions at certain level lead to skeletal fluorosis [3]. As indicated by the World Health Organizations (WHO), the highest admissible limit of fluoride ions is 1.5 mg/L in drinking water while the acceptable fluoride ions concentrations lies in the scope of 0.5 - 1.5 mg/L according to the APHA models [4].
Several physiochemical techniques, such as adsorptions, biodegradations, electrochemical degradations, ions exchange, precipitations coagulations, have been applied to remove fluoride ions from aqueous systems [5]. Among these technologies, adsorptions technology is the most cost effective, eco-friendly and very simple [6] and hence there is need for continued research in the area. Among all types of conventional and non-conventional adsorbents, there has been an increase in the use of numerous waste materials like flyash [7], wood ash [8], tea ash [9], different coal-based adsorbents (coke, bituminous coal, Lignite, fine,) [10], bagasse and cotton jute carbon [11], rice husk, egg shell powder [12], as adsorbents for removal of fluoride ions from aqueous solutions.
Since silicon nitride is known to adsorb some organic compound such as polyacrylate (PAA) and some amino acids, the surface groups can acquire charge in aqueous solution according to the following equations:
\[SiOH{\rm{ }}Si{O^ - }{\rm{ }} + {\rm{ }}{H^ + }\]
\[S{i_2}{\rm{ }}NH{\rm{ + }}{H^ + } \to S{i_2}{\rm{ }}NH_2^ + \]
The electrical charges providing electrostatic stabilization in aqeous suspension promoting the development of a double layer. The strong surface reaction on Si3N4 with H+ and OH- in water involves different kind of interactions, such as the formation of silica and silanols, ammonia and amine related reactions and dissolution and re-adsorption of impurities. The dominant species resulting from these reactions are SiOH2+ and Si2 NH2+ for pHs lower than the IEP; and SiO- and Si2 N- for pH higher than the IEP [13]. It is anticipated that it should be able to adsorb and remove fluoride from water, because of the positive charge on silamine. This study will be the basis for extend research into the use of local material in the synthesis of silicon nitride for water treatment for local consumption in areas with contaminated water due to industrial activities and ground water, using rice husk as source of silica and sugar cane bagasse as source of carbon, thus increasing availability of clean water to both rural and urban areas.
Fluoride ion stock standard solution of 1000 mg/L was obtained by dissolving 2.210 g of anhydrous sodium fluoride (Merck) and diluted to 1 litre in 1000 mL volumetric flask using distilled water. A working solution of 100 mg/L was prepared from the stock by placing 10 ml of the stock solution in a 100 mL volumetric flask and making it to the mark using distilled water. Working fluoride ion standard solutions of 0.1, 2, 4, 6, 8 and 10 mg/L were prepared by adding 0.1, 2, 4, 6, 8 and 10 ml of the working solution into a 100 mL volumetric flask and diluting it to the mark using distilled water.
Fluoride ions were analyzed using a calibrated Fluoride ISE Combined (Sentek, 48030/0001). General operating instructions of optimum pH range: pH 4 to pH 8, temperature range: 0 to 80°C and preconditioning time: 5 minutes were maintained [14]. The recommended ISAB was prepared and mixed with sample solution in the volume ratio of 1:1 to ensure that all measured solutions have the same ionic strength and avoid errors due to differences between the measured activity and the actual concentration. A constant agitation was maintained so as to allow a fresh supply of ions to be exposed to the sensing portion of the ISE. Magnetic stirrers can generate sufficient heat to change the solution temperature. To counteract this effect, a piece of Styrofoam sheet was placed between the stirrer and the beaker to act as an insulating material. The electrode tip was rinsed and gently dabbed dry with a low-lint laboratory tissue between measurements using distilled water to ensure accurate readings.
Approximately twenty five grams of rice husk ash were soaked in HCl for 3 hours, 5 grams of sugarcane bagasse ash and 150mL of 28% ammonium hydroxide was added to the mixture. Teflon was wrapped around the threads before the bomb was tightly closed. The bomb was the heated at various temperature in a thermostat-controlled oven. SNA, SNB, SNC and SND were treated at different temperature of 100, 150, 200 and 300°C for the same period of 24 hours. The contents are allowed to cool and then the resulting residue filtered using whatman No 1. Filter paper. The solid residue, silicon nitride B and C were washed with distilled water.
The flasks, along with test solution and adsorbent, were shaken in Electric shaker (DKZ-1 NO.1007827), to study the various parameters. At the end of desired contact time, the conical flasks were removed from the shaker, and allowed to stand for 5 min for the adsorbent to settle. Subsequently, samples were filtered using Whatman No. 1 filter paper and filtrate was analyzed for residual fluoride concentration. Batch study was conducted to determine the optimum conditions. The effects of pH, adsorbent dose, and temperature, shaking speed, contact time and initial fluoride concentration, on adsorption were studied by varying the parameter of interest and keeping others constant. The effect of pH on fluoride was studied by adjusting the pH of test solution by addition of 0.1 M nitric acid or 0.1 M sodium hydroxide drop wise until the desired pH is obtained.
The effect of pH on removal of fluoride was studied in triplicate by varying pH from 2.0 to 12.0 using adsorbent dose of 0.1g/100 mL. The temperature of the solutions was maintained at 25°C, shaking speed at 120 rpm, and contact time at 100 minutes and fluoride ion concentrations at 6 mg/L. The results are illustrated in Figure 1. The percentage removal of fluoride ion by SNA ranged from about 56.5 to 50.0%, while that of SNB ranged from 59.0 to 54.0%, between pH 2 and 12. The percentage removal by SNC ranged from about 56.0 to 53.0 while that of SND varied from 63.5 to 61.0% between pH 2 and 12. This shows that pH has little effect on the percentage removal of fluoride ions from contaminated water. It is however clear that the optimum pH values for batch adsorptions defloridation experiments were 2 for SNA, SNB, SNC and 4 for SND.
Figure 1: Effect of pH on the removal fluoride ions using SNA, SNB, SNC and SND
There is increase in the percentage amount of fluoride ions adsorbed when increased from 63.5% to 64.6% when the pH was varied from 2 to 5 for SND as shown in graph (Figure 1). As the pH was increased from 5 to 12, the amount of fluoride ions adsorbed by the SND decreased from 64.6% to 61.8% which is statistically significant. The minimum amount of adsorbed fluoride ions occurred at pH of 12. Because of higher H+ ions concentrations at lower pH, the negative charges on the adsorbent surface get neutralized. This in turn reduces the hindrance to diffusions of negatively charged fluoride ions and gives rise to the more active surface for adsorptions. The same trend was also observed in the study done by Basu et al. [4] on the Batch adsorptions of fluoride ions onto microwave assisted activated carbon derived from Acacia Auriculiform is scrap wood, as pH values increased from 2 to 4.4. It may be due to the fact that initially at lower pH, the formations of weak hydrofluoric acid took place which retards the rate of fluoride adsorptions.
For SNA, SNB, SNC, as the pH increases, there was decrease in the amount adsorbed as shown in the Figure 1. The highest adsorptions values were observed at pH 2, where the overall surface charged on the biosorbent may be positive. This empowered the binding of negative charged fluoride ions. At lower pH values, the surface of the adsorbent ended up being positively charged and this encouraged sorption of fluoride particles, likely by the anionic exchange sorption. At lower pH because of the protonation, impact of surface functional groups, such as, thiol, carboxyl, and amino, confers positive charge at surface [15]. Relative sorption inhibitions observed at basic pH range is attributed to the increase of hydroxyl ions leading to formations of aqua-complexes; thereby, retarding the sorption [16].
The impact of dose on fluoride ions adsorptions was investigated using adsorbent materials SNA, SNB, SNC and SND at different adsorbent dose values ranging from 0.2 to 1.0 g. Temperature was maintained at 25°C, shaking speed at 120 rpm, pH at 2 for SNA, SNB, SNC and 5 for SND, contact time was 100 minutes, and fluoride ion concentrations 6 mg/L for SND and 2 mg/L for SNA, SNB, SNC. The results are displayed in Figure 2.
Figure 2: Effect of adsorbent dose on removal fluoride ions using SNA, SNB, SNC and SND
The percentage removal of fluoride ions by SNA and SND gradually increased from 74.8 to 88.2% and 69.0 to 80.0% at dose of 0.2 to 1.0g which is not significant. Percentage fluoride ions removal by SNB ranged from 77.8 to 81.2% at dose of 0.2 to 0.6 g respectively and optimized at adsorbent dose of 1.0g which achieved 81.75% removal. At 0.2 to 0.6 g, removal percentage by SNC is 71.2 to 75.2% which is quite significant and attained optimum at dose of 1.0g with 80.2% removal.
As shown in (Figure 2), percent removal increased to an optimum value with increase in sorbent dosage. It has been observed that up to certain level of higher doses of adsorbents, it resulted into higher removal of fluoride. This is a direct result of higher accessibility of surface and pore volume at higher measurement of the adsorbent. The increasing dosage increases surface range and active destinations accessible for adsorptions of fluoride ions. This increment in adsorptions with dosage can be ascribed to expanded surface zone and the accessibility of more binding destinations for adsorptions [17]. This likewise relate to investigations of Gupta et al. [18] on batch and column operations for the removal of fluoride ions from aqueous solutions using fly bottom ash, that fluoride ions uptake was low at low bottom ash dose and expanded with dosage. This is due to a low dose, all types of sites are entirely exposed and the adsorptions on the surface are saturated faster, showing a higher qe value. However, at a higher adsorbent measurement, the accessibility of higher energysites diminishes with bigger portions of lower energy destinations occupied, bringing about a lower qevalue [18].
However, it can be seen from SNA and SND that there was no significant difference in rate removal of fluoride. It was because of the overlapping of active destinations at higher measurements, consequently decreasing of the net surface regions [19]. This compares to [20], discoveries which reported that expanding the adsorbent measurement relatively builds the rate expulsions of fluoride ions until an ideal quality. A dispersions coefficient KD mirrors the coupling capacity of the surface for a component. The KD estimations of a framework for the most part rely on upon pH and sort of surface.
Adsorptions of fluoride ions were studied using SNA, SNB, SNC and SND. The contact time was varied from 20, 40, 60, 80 and 100 minutes. Temperature was maintained at 25°C, shaking speed at 120 rpm, adsorbent dose of 0.1 g, pH at 2 for SNA, SNB, SNC and 5 for SND, and fluoride ions concentrations 6 mg/L for SND and 2 mg/L for SNA, SNB, and SNC. This is presented in Figure 3.
Figure 3: Effect of contact time on the removal of fluoride ions using SNA, SNB, SNC and SND
The percentage removal of fluoride by SNA and SNB ranges from 75.6 to 82.0% and 76.0 to 82.0% while removal by SNC and SND range from 80.2 to 82.0% and 60.0 to 73.0% between 20 to 100 minutes respectively. This shows that beyond 20 minutes, most Fluoride ions have been adsorbed and contact time has little effect on percentage removal of fluoride ions in contaminated water, because the increase was not significantly different.
The percentage removal also increased as contact time increased. However, it gradually approached an almost maintained value, revealing attainment of equilibrium. Overall removal of fluoride ions for SND occurred in three separate stages as showed in Figure 3. The initial stage showed the harmony stage in which the sorption uptake was generally little. The second stage assigned the moderate uptake of fluoride ions that demonstrated the utilizations of every single active site over the adsorbents surface and fulfillment of saturations or equilibrium stage. The third peak showed the high sorption uptake of the fluoride ions on to adsorbents. Comparable results trend were reported by Ramanaiah et al. [21] who studied fluoride removal on biomass of Spirogyra sp. The initial quick sorption maybe because of functional groups, and active surface destinations on adsorbents surfaces. Presence of such surfaces and functional groups on biomass based adsorbents and initial quick sorption was accounted for by different scientists [16].
Increment in adsorptions with contact time can be attributed to increase surface regions and the accessibility of all the more binding destinations for adsorptions. It can likewise be because of the dispersions of fluoride ions into the surface pores of the adsorbents. In SNA, after sometimes, the increase was less due to the migrations of fluoride ions from upper adsorbent surface to inner pores [22]. As time increases, there is less and less number of adsorptions sites remaining hence reductions in increase in rate of adsorptions. This could have been attributed by the difference in pore sizes of the adsorbents since the larger the pore sizes, the larger the adsorptions capacity [23]. SNC shows that there was equilibrium from 20 to 80 minutes, and this can be attributed to decrease in binding site as the contact time increases.
Temperature influences the adsorptions rate by adjusting the molecular connections and the solvency of the adsorbate [24]. Adsorptions studies were done at different temperatures (20, 40, 50, 60 and 80°C) using adsorbent material SNA, SNB, SNC, SND of 0.1 g, shaking speed of 120 rpm, contact time of 100 minute, pH 5 for SND, 2 for SNA, SNB, SNC, initial fluoride ions concentrations of 6mg/L for SND, 2 mg/L for SNA, SNB, SNC. The result is illustrated in Figure 4.
Figure 4: Effect of temperature on removal of fluoride ions using SNA, SNB, SNC and SND
It was observed that Adsorptions of fluoride ions decreased from 62.0% to 54.0% when the temperature was increased from 20°C to 80°C for SND, percent removal when SNA was used with varying temperature decreased from 79.0% to 66.5% at temperature of 20 to 80°C, when SNB was used the adsorptions rate decreased from 81.2% to 76.8% at temperature of 20°C to 80°C, while using SNC, the adsorptions rate decreased from 80.8% to 61.0% at temperature of 20°C 80, respectively.
These results indicate that high temperatures work against defluoridations by silicon nitride. This is on the grounds that with increasing temperature, the attractive force between adsorbent surface and fluoride ions are weakened and the sorption diminishes. Thus, at 20°C highest amount of fluoride has been removed. This outcomes are in concurrence with Zhao et al. [25], examinations on fluoride removal from aqueous solutions using synthesized Fe3 O4 -Al(OH)3 attractive nanoparticles. The adsorptions capacity decreased with temperature. At high temperature, the thickness of the limit layer decreases because of the high propensity of the fluoride ions to escape from the adsorbent surface to the arrangement stage which results in a lessening in adsorptions as temperature increases [26]. Increase in temperature increases the rate of diffusions of the sorbate ions towards the sorbent surface and at the same time, some sorbate ions acquire enough thermal energy and diffuse away from the monolayer surface.
This implies that the rate of desorption was higher than the rate of adsorptions, which means that adsorptions is an exothermic reactions [27]. The reductions in the measure of fluoride ions adsorbed with temperature shows an exothermic nature of the process. It is clear that the temperature of the adsorptions medium could be important for energy dependent mechanisms in fluoride ions binding process.
The agitation of fluoride solutions was carried out in an electric shaker at ambient temperature. The agitations speed varied from 120, 240, 360, 480 and 600rpm. Temperature was maintained at 25°C, contact time of 100 minutes, adsorbent dose of 0.1 g, pH at 2 for SNA, SNB, SNC and 5 for SND, and fluoride ions concentrations 6 mg/L for SND and 2 mg/L for SNA, SNB and SNC. The result is presented in Figure 5.
Figure 5: Effect of shaking speed on removal of fluoride ions using SNA, SNB, SNC and SND
Fluoride uptake increased with the increase in shaking rate. Percentage removal of fluoride ions increase is statistically significantly from 61.0% at shaking speed of 120 rpm to 62.0, 63.0 and 64.0% at a shaking speed of 240, 360 and 480 rpm for SND. Percentage removal of fluoride ions increase is statistically significantly from 76.5% at shaking speed of 120 rpm to 78.0, 79.5 and 79.8% at a shaking speed of 240, 360 and 480 rpm then attained maximum percent removal at 80.5% at 600 rpm when adsorbent SNA was used. . For SNB, 79.2% was recorded at shaking speed of 120 rpm which increased to 79.8, 80.5, 80.5 and 81.5% at shaking speed of 240, 360, 480 and 600 rpm. Furthermore, when SNC was used, percentage removal of fluoride ions changes from 78.75% at shaking speed of 120 rpm to 78.8, 81.0, 81.5 and 81.5% at shaking speed of 240, 360, 480 and 600 rpm respectively. For SNB and SNC, the increase in percentage removal is not statistically significantly. The optimum shaking speed for the removal of fluoride ions using all the adsorbents was 600 rpm
These outcomes can be connected with the way that increase in the unsettling speed enhances the diffusions of fluoride ions towards the surface of the adsorbent, and in this manner, proper contact is produced between fluoride ions in solutions and the binding destinations. This advances exchange of sorbate ions to the sorbent destinations. Higher uptake of fluoride ions could be conceivable at higher rate of shaking as it will guarantee that all adsorptions destinations are made promptly accessible for fluoride ions uptake. This pattern is in concurrence with results reported by Bernard et al. [28].
While expanding the shaking speed, the disseminations rate of ions from the mass fluid to the fluid limit layer encompassing sorbent ions gets to be higher in view of an upgrade of turbulence and decrease of the thickness of the fluid limit layer [29]. Great disseminations of ions towards the sorbent particles are additionally guaranteed. Increasing the agitations rate builds the outside film mass exchange and coefficient consequently the rate of uptake [30]. This is because of decrease of the film limit layer encompassing the sorbent particles.
Awan et al. [22] also observed that at lower rpm, the removal capacity was low but as the rpm increased, the fluoride removal also increased, and this might be due to the better contact between adsorbate and adsorbent at high rpm. Increase in kinetic energy increases the rate of diffusions of fluoride ions hence more fluoride ions reach the adsorbent surface thereby increasing the rate of both physisorption and chemisorption [31]. If shaking speed is low, silicon nitride accumulates instead of spreading in the sample and various active sites are buried under the outer layer reducing the rate of adsorptions. This indicates that shaking speed should be sufficient to ensure that all the surface binding sites are available for fluoride uptake.
The experiments were carried out using 100 mL of analyte solutions with concentrations varying from 2 to 10 mg/L at pH 6. For adsorbent material SNA, SNB, SNC, SND an amount of 0.1 g/100 mL of adsorbent was used for each adsorptions experiment, shaking speed of 120 rpm, temperature of 25°C and contact time 100 minutes. The results are shown in Figure 6.
Figure 6: Effect of initial concentrations on removal of fluoride ions using SNA, SNB, SNC and SND
As the initial concentrations increased from 2 mg/L to 6 mg/L, there was an increase in the percentage quantity of fluoride ions adsorbed by the adsorbent from 62.5% to 65.0%, increase in initial ions concentrations above 6 mg/L was accompanied by a decrease in percentage defluoridations by using SND, The percent removal of fluoride was about 75.0% at initial concentrations of 2 mg/L and then reduced to 54.0% at 10 mg/L when using SNA. For SNB, the percentage removal dropped from about 72.0% for initial concentrations of 2 mg/L to 54.0% at 10 mg/L, while when using SNC, the percentage removal of fluoride decreased from about 66.0% for initial concentrations of 2 mg/L to 56.8% at 10 mg/L.
The increase in percentage removal by using SND from 62.5 to 65.0% is probably due to larger surface area of the SND being available at beginning for the adsorptions of fluoride ions. The decrease in percentage removal above 6 mg/L is on account of the surface adsorptions destinations get to be depleted; the uptake rate was controlled by the rate at which the adsorbate was transported from the outside to the inside sites of the adsorbent particles. The highest adsorptions rate of fluoride particles happened at a fundamental molecule gathering of 6 mg/L. At this underlying fluoride particle fixation, 65.0% of the underlying grouping of fluoride particles was adsorbed. Introductory particle convergence of 6 mg/L was recorded as the ideal focus and utilized as a part of all fluoride adsorptions tests where different parameters were varied for SND. There was an expansion in adsorptions because of an increment in the measure of the fluoride particles bound on the surface of the adsorbent. Lvet al. [32] acquired a comparative pattern in their study on fluoride particle expulsions with MgAl-CO3 . As per Malakootian et al. [33], expulsions of fluoride particles from fluid arrangement by pumice demonstrated an expansions in adsorbed rate as beginning fluoride fixations was expanded because of a bring up in the main impetus of the focus slope.
When using SNA, SNB and SNC, the percentage removal decreases as the initial concentrations increases, because the adsorbent materials get depleted forcefully with the expansions in initial fluoride concentrations. This was because of the way that for an altered adsorbent measurement, the aggregate accessible adsorptions sites were constrained, which got to be soaked at a large concentrations. Comparative pattern has been accounted for fluoride removal by utilizing charcoal of a Neem [34]. The contrast between the mean rate removal for SNA, SNB, SNC and SND varied essentially as the concentrations of fluoride increased. This could be due to the distinctions in pore sizes and level of crystallinity of the adsorbents. Adsorbent with bigger pore sizes are preferred over those with smaller pore sizes. The higher the level of crystallinity, the better the spreading of pores making them more accessible for adsorptions forms. Several researchers have found that there is a decline of removal of fluoride ions with increase of initial concentrations [35].
The Freundlich et al. [36] and Langmuir [37] models are the most utilized models in adsorptions studies. Equilibrium data acquired were fitted to the Langmuir and Freundlich isotherms. Thermodynamic information of fluoride adsorptions using SNA, SNB, SNC and SND are presented in Table 1.
|
Langmuir isotherm |
Freudlich isotherm |
|||||
ions |
Adsorbents |
Qmax (mg/g) |
b (dmg3/g) |
R2 |
1/n |
k |
R2 |
F- |
SNA |
7.8554 |
0.4829 |
0.9888 |
0.5447 |
2.4468 |
0.9531 |
SNB |
8.9454 |
0.3450 |
0.9977 |
0.6189 |
2.2299 |
0.9737 |
|
SNC |
13.9 |
0.1534 |
0.9992 |
0.7943 |
1.8213 |
0.9981 |
|
SND |
49.75 |
0.0352 |
0.9792 |
0.8561 |
1.7697 |
0.9455 |
Table 1: Thermodynamic information of fluoride adsorptions using SNA, SNB, SNC and SND
Qmax= adsorptions capacity of Langmuir, b= Langmuir equilibrium constant associated with energy of adsorptions, R2 =Correlations coefficient, 1/n = heterogeneity parameter and kf = adsorptions capacity of Freundlich.
The adsorptions data of fluoride ions on SNA, SNB, SNC and SND fitted best into Langmuir adsorptions isotherm model based on the R2 values of 0.9888, 0.9977, 0.9992 and 0.9792 as shown in Table1 and Figures 7-10 respectively. This implies that the Langmuir adsorptions isotherm has higher values of R2 hence it fits well to the experimental data obtained than the Freundlich adsorptions isotherm, for fluoride ions. This indicates that the adsorptions of fluoride ions on the SNA, SNB, SNC and SND are monolayer. The value of b indicates the adsorption nature to be unfavorable if b>1, linear if b=1, favorable if 0<1, and irreversible if b=0, therefore, the values indicate that Langmuir isotherm is favorable. The Langmuir b values for SNA, SNB, SNC and SND were 0.4829, 0.3450, 0.1534 and 0.0352 indicating that the values decreased as the temperature of the hydrothermal synthesis increased. The adsorptions capacities of SNA, SNB, SNC and SND were 7.9, 8.9, 13.9, 49.8 mg/g. This implies that the adsorptions capacity increases as the temperature increases. The adsorptions capacity values were higher than those of adsorbents reported in Table 2. This suggests adsorbent SND is preferred adsorbents over the reported adsorbents. This is on the grounds that most adsorbents contain smaller scale pores and the adsorptions procedure is influenced by surface properties, for example, surface regions and extremity. A large surface zone is best to provide huge adsorptions capacities. The measure of the miniaturized scale pores decided the openness of the sorbate atoms to the adsorptions surface [38].
Figure 7: Langmuir adsorption isotherm for fluoride ion using SNA at 25°C
Figure 8: Langmiur adsorption isotherm for flouride ion using SNB at 25°C
Figure 9: Langmuir adsorption isotherm for fluoride ion using SNC at 25°C
Figure 10: Langmuir adsorption isotherm for fluoride ion using SND at 25°C
Adsorbate |
Adsorbent |
Adsorptions capacity(mg/g) |
Reference |
Fluoride ions |
Rice |
0.019 |
Wahecdet al., 2009 |
Ground nut shell |
2.32 |
Veeraputhiran etal., [20] |
|
CynodonDactylon |
4.617 |
Alugumuthu et al., [20] |
|
Acacia Auriculiformis |
18.95 |
Basu et al., [4] |
Table 2: Comparison of monolayer adsorptions capacity of silicon nitrides (SNA, SNB, SNC and SND) with other adsorbents for fluoride
Regarding Freundlich isotherm, the slope 1/n ranging between 0 and 1 is a measure of adsorptions intensity or surface heterogeneity, becoming more heterogeneous as its value gets closer to zero. A value for 1/n below one indicates a normal Langmuir isotherm while 1/n above one is indicative of cooperative adsorptions and it also implies that the adsorptions is favorable. 1/n Values of 0.5447, 0.8561, 0.7943 and 0.8561 were observed for fluoride ions for SNA, SNB, SNC and SND respectively indicating a normal Langmuir fit.
Adsorbent SNA, SNB, SNC and SND are good adsorbent of fluoride, based on their percentage removal registered when initial ion concentration, pH, contact time, shaking speed, temperature and adsorbent dose varied.
SND was a better adsorbent than other adsorbents, this conclusion was derived based on adsorption capacities of fluoride attained on the adsorbent.
The present study shows that the Silicon nitride can be employed as a potentially low-cost sorption for removal of fluoride ion from waste water. Their adsorptions were found to be greatly dependent on the initial concentration, shaking speed, contact time, temperature of the solution.
I sincerely thank the Water Resource Management Authority (WRMA) for allowing me to carry out part of my study in their central laboratories. I also take this opportunity to thank the Kenyatta University Chemistry Department staff and laboratory technicians for their support during my research.
Download Provisional PDF Here
Article Type: Research Article
Citation: Abdulhameed A, Mbuvi HM, Changamu EO (2017) Removal of Fluoride Ion by Silicon Nitride Powder. Int J Water Wastewater Treat 3(1): doi http://dx.doi.org/10.16966/2381-5299.137
Copyright: © 2017 Abdulhameed A, et al. This is an open-access article distributed under the terms of the Creative Commons Attribution License, which permits unrestricted use, distribution, and reproduction in any medium, provided the original author and source are credited.
Publication history:
All Sci Forschen Journals are Open Access