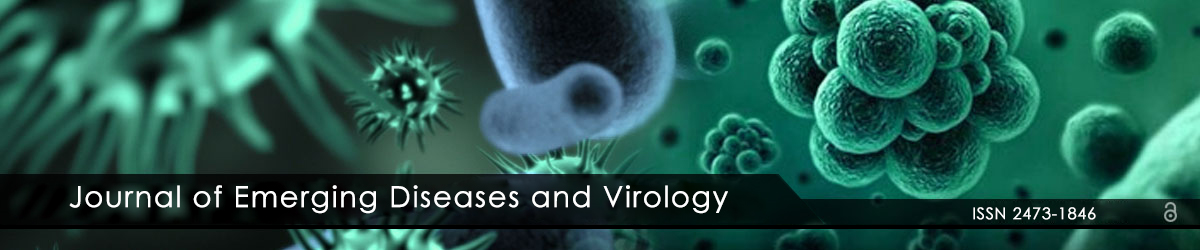
Full Text
Stella Rousset1 Martin Gauthier2 Anne Huynh2 Jean-Michel Mansuy1*
1Laboratoire de virologie, Institut Fédératif de Biologie, CHU Purpan, France2Service d’hématologie, IUCT Oncopole, France
*Corresponding author: Jean-Michel Mansuy, Laboratoire de virologie, Institut Fédératif de Biologie, CHU Purpan, France, Tel: +33 5 676 90 418; Fax: +33 5 676 90 425; E-mail: mansuy.jm@chu-toulouse.fr
This immunocompromised patient suffering from severe sapovirus colitis and enteritis became dehydrated and losing weight. He was admitted to our intensive care unit but relapsed after two months of follow-up while on intravenous immunoglobulin maintenance. Sapovirus is becoming an increasingly important pathogen responsible for sporadic and epidemic acute gastroenteritis worldwide, especially in young children. It is usually diagnosed by multiplex RT-PCR analysis of feces, which can differentiate it from other causes of infectious diarrhoea. Sapovirus does not seem to cause more severe infections in immunocompromised patients or to lead to chronic infections due to the emergence of recombinant strains, unlike norovirus, a Calicivirus with several similar features. Its potential for nosocomial epidemics is also still unclear. Most treatment of sapovirus infections focus on the symptoms as the disease is often benign. No specific antiviral treatment is presently licensed. Perhaps the proposed use of immunoglobulins or nitazoxanide to treat norovirus in immunocompromised patients could be extended to sapovirus, but these procedures remain experimental. We suggest that this increasingly important enteric pathogen that can cause severe, prolonged diarrhoea in immunocompromised patients should be closely monitored.
Sapovirus; Diarrhoea; Immunocompromised patient; Intravenous immunoglobulins; Multiplex real-time RT-PCR
A man aged 44 was admitted to the hematology ward of Toulouse University Hospital in April 2017 for autologous stem cell transplantation as treatment of his Hodgkin’s lymphoma. The lymphoma was diagnosed in December 2015, with lung and colon involvement (stage IV in Ann Arbor staging system). It had relapsed after first-line chemotherapy with doxorubicin, bleomycin, vinblastine and dacarbazine, but was in complete metabolic response after a second-line treatment with bendamustine and brentuximab in June 2016. The proposed therapy was to prolong the response by performing two successive autologous stem cell transplantations. The patient was also suffering from denutrition (body mass index 18.5 kg/m2), frequent lower respiratory tract infections, pulmonary pneumocystosis in December 2016, plus an episode of Clostridium difficile colitis in February 2017.
The patient had been suffering from frequent liquid diarrhoea for several weeks, with no other intestinal symptoms (abdominal pain, nausea or vomiting) or fever when he was admitted. Multiplex RT-PCR for enteric viruses performed on stools revealed the presence of sapovirus. Stool analyses were otherwise negative for enteropathogenic bacteria (Salmonella, Shigella, Campylobacter, E coli) and Clostridium difficile. He was initially treated with 20 g/ day Intravenous Immunoglobulins (IVIg). Induction chemotherapy (carmustin, cytarabine, etoposide and melphalan) was begun on April 14 and autologous stem cell transplantation was performed on April 20. The first day of chemo-induced aplasia was April 21.
His diarrhoea became worse, he became feverish, and his abdomen became distended and tender. Blood tests showed hypokalemia and hypomagnesemia requiring intravenous supplements. He also developed hypoalbuminemia and hypogammaglobulinemia that was refractory to IVIg supplements, evoking exudative enteropathy. A CTscan showed signs of enteritis and sigmoidal colitis. The patient did not respond to broad-spectrum antibiotics (meropenem, vancomycin, amikacin, ciprofloxacin, and metronidazole) or antifungal treatment (caspofungin). He was transferred to the intensive care unit on April 26 suffering from septic shock, hypotension (mean arterial pressure 50 mmHg) and tachycardia (140 bpm). The patient was repeatedly given intravenous fluid resuscitation and antibiotics.
Subsequent stool analyses (April19, May 16, and May 23) were still positive for sapovirus alone. Concomitant infections included an upper respiratory tract rhinovirus infection, Staphylococcus haemolyticus pneumonia, Epstein-Barr and BK virus-induced hemophagocytic lymphohistiocytosis that were treated with dexamethasone and IVIg.
Analysis of sigmoidal biopsies obtained by a short colonoscopy performed on May 12 showed structural distortions of the colonic mucosa (atrophic glands, hollow chorion), but no active inflammatory lesions or viral cytopathic effect. Immunohistochemical studies on the colon biopsy with anti-CMV antibody, CMV PCR and sapovirus PCR were all negative.
His condition slowly improved; the fever and diarrhoea abated after a second course of intravenous immunoglobulins on May 12th and progressive enteral renutrition via a naso-gastric drip. Antibiotics were gradually stopped after treatment for 21 days. The patient was then returned to the hematology ward and discharged on May 29th after resolution of his chemo-induced aplasia. The patient still had some symptoms at discharge, including episodic diarrhoea and abdominal discomfort. We proposed monthly anti-infectious intravenous prophylactic perfusions of immunoglobulins.
Diarrhoea reappeared two months later in early July 2017, with dehydration and hydro-electrolytic disturbances, needing admission to a medical ward. Stool analysis performed on August 1st, 112 days after the first stool analysis, remained positive for sapovirus. As before, there were no other enteropathogens. The patient’s evolution was unfortunately unfavorable. He deceased on September 28th after a progressive deterioration of the general state, in cachectic condition with cytopenias and multiple bacterial infections (blood, urinary and lower respiratory tract).
Virology
Sapovirus is responsible for acute gastroenteritis in humans and animals. It belongs to the Caliciviridae family (together with norovirus, lagovirus, nebovirus, and vesivirus). The Sapovirus genus, Sapporo virus species are described in the report of the International Committee on the Taxonomy of Viruses 2016 [1]. Sapovirus particles were first observed by electron microscopy of human stool samples in 1976. These small (30-38 nm diameter) icosahedral particles have cupshaped depressions on their surface, something like a star of David. The virus is unenveloped, making it resistant in the environment. The prototype strain, Hu/SaV/Sapporo/1982/JPN, was isolated from the Sapporo gastroenteritis outbreak in an orphanage in 1982: hence its name. While there are animal sapoviruses, zoonotic infection is not possible because there is an interspecies barrier [2-4]. Although a porcine sapovirus cell culture has been developed in a murine model, no human sapovirus cell culture model is yet available, limiting our knowledge of its replication cycle [5].
The virus genome is composed of one 7.1-7.7 kb long positivesense, single RNA strand. The first complete genome sequence was determined for the Manchester strain Hu/Manchester/93/UK in 1993 and the full-length genome determined later was found to be 91.7% homologous to that of the Manchester strain [6]. It contains 3 distinct Open Reading Frames (ORF). ORF1 encodes a long polyprotein that is processed by the virus protease to give 6 nonstructural proteins and the major capsid protein VP1 [7,8]. ORF2 is predicted to encode the minor structural protein VP2, while the function of ORF3 in human strains remains unknown. Key enzymes involved in virus replication are the RNA-dependent RNA polymerase (RdRp) [9] and the RNA helicase eIF4A [10]. The major capsid protein VP1 is essential for the assembly of virus particles [11,12]. The VP1-encoding region is the most diverse one, as it includes a Center Variable Region (CVR). Sapoviruses are divided into genogroups and genotypes according to their VP1 genetic sequences [13]. Four human sapovirus genogroups (GI,GII,GIV,GV) have been defined, whereas genogroup III infects pigs. Antigenicity differs among genogroups and genotypes, suggesting that the VP1 sequence is linked to virus antigenicity [14-16]. The other regions (the RdRp-region and the RdRp-VP1 junction region) are less diverse than VP1, thus less relevant for establishing a reliable classification. However they may be useful for epidemiological surveys and the characterization of recombinant strains.
Recombinant strains are defined as sapoviruses with inconsistent grouping between VP1 and the RdRp region. Recombination may occur between genogroups, or genotypes belonging to the same genogroup. The sequences of both VP1 and RNA polymerase are needed to identify recombinant viruses [17,18].
The sapovirus geno groups and genotypes in a given geographical area evolve over time [19-22]. This time-evolution pattern is probably due to the genetic diversity induced by mutations triggered by RNA polymerase activity and their selection [21]. The coexistence of several predominant strains during epidemics favors the emergence and stabilization of recombinant strains in a single geographical area [23]. Human-human transmission occurs via the fecal-oral pathway (either directly via contact with infected feces, vomitus, or indirectly via surfaces), or by consumption of contaminated food (especially shellfish) or water [24-26]. Sapovirus can be a public health problem as it causes outbreaks notably in health care settings, and sporadic cases worldwide. They are facilitated by a low infectious dose (1500 to 2800 genomic copies), the shedding of large amounts of virus, great environmental stability and relative resistance to disinfectants. Sapovirus is one of the most frequent agents causing acute gastroenteritis [27-31]. Outbreaks occur in people of all ages and throughout the year, unlike sporadic cases, which mostly affect children less than 5 years old, with a seasonal peak in winter [32]. Outbreaks are especially likely to occur in semiclosed settings: childcare centers, schools, hospitals, long-term care facilities, restaurants, hotels, etc. [33-36]. Sapovirus was detected in 1.3 to 22.6% of stool samples taken during gastroenteritis outbreaks [4,22, 32,37,38]. To date, the largest food-borne sapovirus outbreak occurred in Japan in 2010; it was spread by lunch boxes handled by people working in a single catering company [39]. Coinfections with other enteric viruses (adenovirus, norovirus, etc.) or other sapovirus genogroups/genotypes have been reported [24,40,41].
No host susceptibility factor to sapovirus has yet been identified. Susceptibility to human sapovirus is not associated with histoblood antigen phenotypes, unlike sensitivity to norovirus [42]. Malnourishment and poor sanitary conditions may also be susceptibility factors [43]. The replication sites within the host, the pathological changes induced by sapovirus, and the mechanisms underlying immunity to it are yet to be characterized. Humoral protection may be involved as mother-to-child protection was reported during an outbreak in a mother/infant unit [44]. Seroprevalence reaches 90% in children and adults, suggesting that childhood sapovirus infections are common [45]. Studies using a porcine sapovirus cell culture model indicated the involvement of innate immunity factors, particularly type I interferon [46]. Protection seems to be genogroup and genotype-specific, as reinfections with different genogroup/genotype sapoviruses can occur [19,32].
Clinical presentation
Sapovirus is responsible for acute gastroenteritis (liquid diarrhoea, vomiting, abdominal pain), with usually no fever, unlike bacterial diarrhoea. The mean incubation period is 1.7 days (less than 1 to 4 days) [47]. Other cardinal symptoms include fatigue, chills, myalgia, and headache. Rare complications include intussusception [48], and severe abdominal distension that can be mistaken for occlusive syndrome [49]. Some people can be asymptomatic while still shedding great virus loads in their stools [4,39,50]. The disease is rapidly selflimited and is resolved within 1 week in immunocompetent patients [33,34,39,51]. Despite improvements in the intestinal symptoms, virus continues to be shed for 1 to 4 weeks after disease onset [52,53]. The gastroenteritis caused by sapovirus is reported to be less severe than that caused by norovirus or rotavirus [51,53]. Nevertheless, it can lead to hospitalization and death, especially in frail, elderly patients during outbreaks in long-term care facilities [34]. It is not yet clear whether sapovirus can cause more severe disease in at-risk patients, as does norovirus in immunocompromised persons and neonates [54,55]. There have been few reports of cases of sapovirus-induced chronic diarrhoea in immunocompromised patients [40,54]. A study on a cohort of 16 renal transplant patients suffering from chronic diarrhoea associated with calicivirus, included 1 patient who was sapoviruspositive; his diarrhoea lasted 147 days and virus continued to be shed into his gut until day 157 [54].
Laboratory diagnosis
Several methods are used to detect sapovirus in human, usually stool, samples [56]. Diagnosis can be direct, by electron microscopy, detecting antigens or nucleic acids. Transmission Electron Microscopy (TEM) is no longer routinely performed in most laboratories. Antigens are generally detected by Enzyme-Linked Immunosorbent Assays (ELISA) [57]. Although they are very specific, these assays are less sensitive than nucleic acid detection, and they cannot detect antigenically diverse sapoviruses. A future challenge will be to develop a pangenogroup/pangenotypic ELISA or immunochromatography test, that uses a panel of broadly reactive monoclonal antibodies, or that targets a VP1 epitope common to all genogroups [58-60]. Serology enables indirect diagnosis, but is not clinically relevant as the seroprevalence in human populations is over 90% [45].
Nucleic acid detection methods are used for the routine detection of enteric viruses in clinical specimens. The most frequently used, realtime RT-PCR, is more sensitive than TEM and ELISA, and is widely available. Other methods include conventional RT-PCR with migration of amplicons by agarose gel electrophoresis and microsphere-based detection of fluorescent PCR products (Luminex technology) [61-65]. Primers may target the VP1 region, the RdRp region or the RdRPVP1 junction region, depending on the test. Most assays include multiple or degenerate primers to cover the great genetic diversity of sapoviruses, but some may still miss particular genogroups [66]. Assays targeting the RdRp-VP1 junction region are the most sensitive and can be used as the first choice for screening clinical specimens for sapovirus. The VP1 region enables virus genotyping. RdRp primers may detect conserved patterns found in other human enteric viruses [56]. Multiplex assays for enteric viruses are the most frequently used in clinical practice. Some tests provide fast detection of viral, bacterial and parasitic enteropathogens at the same time [67-70]. Metagenomics applied directly to stool samples may become a very useful diagnostic tool in the future [71]. Full genome sequencing is not used in routine clinical practice [72].
The test used in this case was a commercial multiplex real-time RTPCR (ALLPLEX™ GI-Virus Assay, Seegene®), that can be used for feces, rectal swabs, and intestinal biopsies. It detects 6 viral targets (norovirus GI, norovirus GII, rotavirus, adenovirus 40 and 41, astrovirus and sapovirus). For sapovirus, genogroups GI, GII and GIV are detected.
Parallel with Norovirus
Sapovirus has several virological, epidemiological and clinical features in common with norovirus, as they both belong to the Caliciviridae family. Like sapovirus, norovirus is a non-enveloped virus with a single-stranded, positive-sense, 7700 nucleotide-long RNA genome. It was discovered in 1972 by electron microscopic examination of feces from a subject who was part of a gastroenteritis outbreak in a school in Norwalk. The genus is made up of 6 genogroups and more than 30 genotypes, with genotype GII.4 being the most prevalent worldwide. Variant strains have been generated by mutations and recombinant strains that contribute to the great genetic diversity of norovirus [73]. There are noroviruses that infect cattle, pigs, and sheep, but transmission is species-specific and thus no zoonotic transmission has been described. While there is no culture model for human norovirus, a murine norovirus culture model has been used to study the virus life cycle and its interactions with the host immune system and microbiota [74].
Norovirus is the main agent responsible for acute gastroenteritis in adults and adolescents in the United States, and the second, after rotavirus, in young children less than 5 years old [75]. Among Nicaraguan children universally vaccinated against rotavirus, norovirus and sapovirus have become the leading causes of gastroenteritis [76]. Similarly to sapovirus, norovirus also causes sporadic and epidemic gastroenteritis. It is transmitted via the fecal-oral route. Its contagiousness is enhanced by the same factors as for sapovirus: low infectious doses (as low as 10 units PCR [77]; resistance in the environment, resistance to heat, low and high pH, and to usual concentrations of sodium hypochloritebased cleaning solutions. A prolonged fecal excretion often observed after recovering also participates to the contagiousness. The globalization of the food industry may favor large norovirus outbreaks [73]. Nosocomial outbreaks are feared, especially in longterm care facilities for elderly people and immunocompromised hosts (transplant units, chemotherapy units), because of the great genetic diversity of the strains involved and the high attack (50%) and transmission rates (27%).
The morbidity and mortality rates due to norovirus are enormous, as is its socio-economic burden, quite unlike those of the much less prevalent sapovirus. It is estimated to cause around 20 million cases, 70.000 hospitalizations, and 800 deaths per year in the USA and its cost to society is estimated at around 60 billion dollars per year [78,79]. Norovirus is responsible for the death of more than 2, 00,000 children per year in developing countries [80]. Global mortality is around 0.8-2.8%, resulting from dehydration, malnutrition, sepsis, inhalation pneumopathy, but rarely from necrotizing enterocolitis, colon perforation, or acute gastro-intestinal bleeding (MalloryWeiss syndrome) [75,81]. Genetic factors make hosts susceptible to norovirus infections. The Histo-Blood Group Antigens (HBGA) includes the ABO group and Lewis group antigens, and harbour a genetic polymorphism. Their genes encode glycan molecules (heparan sulfate, sialic acids, β-galactosyl ceramide) that are used by the virus to bind to and enter small intestine enterocytes, with each norovirus strain having a characteristic HBGA-binding profile. The HBGA are thus a target for developing an antiviral treatment [82].
The clinical picture is similar to that for sapovirus. Disease occurs after a short, 24-48 hour, incubation period [47]. It is mild and self-limited in immunocompetent hosts, and symptoms resolve in around 3 days. However, norovirus may cause chronic infections in immunocompromised hosts [83,84] (people with congenital immune deficiencies [85], HIV-infected hosts [86,87], cancer patients [88], people with hemopathies [88], solid organ transplant recipients [89- 91], allogeneic stem cell transplant patients [88,92,93] and elderly people with comorbidities). The prevalence of norovirus infections in immunocompromised hosts is not well-established: it may be around 17-18% [84]. It represents a challenge in terms of diagnosis (differential diagnosis notably includes graft-versus-host-disease), prevention of transmission (with a risk of nosocomial transmission and outbreaks in hospitals), and therapeutic management. Chronic norovirus infections may last for years in immunocompromised hosts, causing weight loss, graft dysfunction and decreased quality of life; it may also exacerbate the outcome of the underlying disease [54,94]. Asymptomatic healthy hosts may shed virus for weeks or months, and immunocompromised ones for years, raising the possibility of a long-lasting reservoir for future outbreaks and/or the emergence of variant or recombinant strains in individuals [90,95-97]. However, some clinical observations do not favor the emergence of new norovirus strains in chronically infected hosts [98,99]. Treatment is developed later; reducing immunosuppression, is, whenever possible, the therapeutic priority in any chronic norovirus infection, as the B-cell and T-cell responses must recover in order to clear the virus [54,100,101].
Treatment and prevention
No specific antiviral curative treatment for sapovirus is yet available. The key features of treatment are supportive care with rehydration (oral or/and intravenous) and renutrition (sometimes enterally or/ and parenterally in cases of prolonged, severe diarrhoea [100]. The experimental treatments that have been proposed for norovirus gastroenteritis, or prophylactic use in epidemics could also be applied to sapovirus infections, but none has been specifically studied. None of these strategies has been approved in clinical trials, but any that are proved to be efficient and safe could be useful in high-risk patients, including immunocompromised ones. These include:Passive immunotherapy with intravenous polyvalent immunoglobulins [102]. They act as immunomodulators, cause opsonization, and may contain specific anti-sapovirus antibodies.
- Passive immunotherapy with specific monoclonal anticapsid antibodies can neutralize norovirus and prevent infection [60,101].
- Oral immunoglobulins seem to have reduced norovirusinduced diarrhoea in one retrospective study on immunocompromised patients, but the difference was not statistically significant. No benefit was found in terms of time in hospital or cost [103].
- Drugs targeting key factors of the virus cycle. Research has identified compounds that inhibit norovirus replication in vitro, some of which are also effective in vivo in murine models. RdRp polymerase inhibitors [9], protease inhibitors, and putative NTPase/ helicase inhibitors [10] can all target specific virus proteins. The antiviral properties of interferon can be used against norovirus [46]. Novel strategies include targeting essential host cell factors, or exploiting the high mutation rate of noroviruses (ribavirin and favipiravir seem to possess lethal mutagenesis properties) [104-106]. The great genetic variability of these viruses, due to the imprecision of the viral polymerase during replication and the potential for strains to recombine, is a barrier to the development of potent drugs against norovirus and sapovirus. An efficient therapy should anticipate the risks of virus evasion by acting against all genotypes and having a high barrier to resistance. The ideal antiviral treatment should therefore combine at least two drugs whose resistance profiles do not overlap, to decrease the risk of resistant variants emerging in chronically infected patients and in outbreaks [107].
- The anti-parasitic, antiviral thiazolide drug nitazoxanide produced a modest decrease in symptom duration in healthy individuals, and resolved the symptoms in one patient chronically infected by norovirus [108].
- A recent drug screening seemed to indicate that theaflavins have an antiviral effect against Caliciviridae [109].
Global measures for preventing virus transmission between individuals rely on scrupulous hygiene: frequent hand-washing or the use of hand sanitizers; isolation of sick patients, especially in longterm care facilities (sick employees should respect hygiene advice even long after resolution of symptoms, as virus may be shed for several weeks); decontamination of surfaces with 0.5% sodium hypochloritebased cleaning solutions. Most environmental prevention relies on monitoring wastewater stations and prohibiting wild rejections. RTPCR monitoring of food production and water areas (notably shellfish farms), has been proposed to accelerate public health alerts, but the threshold for clinical symptoms and alerts, and the pertinence and cost/benefit ratio of this strategy, remain to be evaluated [110-113].
There is currently no vaccine against sapovirus. Most research is focused on finding a curative and/or preventive vaccine effective against norovirus. Creating a vaccine must overcome problems of virus diversity and incompletely understood characteristics such as virus-host cell binding and duration of immunity after infection. Identifying important capsid VP1 antigenic sites, developing improved model systems and further studies in humans will all help to design future vaccines [58]. Multivalent vaccines based on virus particles may have cross-protective activity, and seem promising in terms of immunogenicity; they could be available within 10 years. Target populations are those at risk of severe disease (immunocompromised patients and the elderly) and those at risk of propagating disease (e.g., healthcare workers); further studies will help determine the best ways to protect and prevent transmission among these groups [114,115].
To our knowledge, this is the first case report of life-threatening acute gastroenteritis associated with sapovirus in an immunocompromised patient. His immunodeficiency resulted from treating his Hodgkin’s lymphoma with induction chemotherapy and autologous stem cell transplantation. The clinical picture was particularly severe as it occurred in a patient with other problems, including Clostridium difficile membranous colitis and undernourishment. His dehydration was severe enough to require massive intravenous fluid resuscitation and hydro-electrolytic supplementation, and his denutrition needed parenteral and enteral feeding; all of which led to his admission to an intensive care unit. The symptoms were not specific for an enteric virus, and undoubtedly several other factors contributed to his intestinal disease: the microbiota dysbiosis induced by using broad-spectrum antibiotics and the recent Clostridium difficile infection, the toxicity of chemotherapy on the gut mucosa, and a possible contribution from neutropenic enterocolitis. There was no evidence that other enteric pathogens were involved.
The diarrhoea and virus shedding continued for longer (at least 112 days) than they would have in immunocompetent patients, which is consistent with published reports. Unfortunately, quantitative PCR is not available in our laboratory; it would have been useful to compare its results to immunocompetent patients suffering from sapovirus gastroenteritis, and to monitor the intensity of virus shedding over time. We are also unable to sequence sapovirus in our laboratory, and therefore do not know its genogroup and genotype, neither could we study any recombinant strains that might have emerged during follow-up.
The diarrhoea that occurs in immunocompromised patients suffering from norovirus infection is known to be more severe and prolonged than that occurring in immunocompetent patients, but the difference with sapovirus is not clear. This case suggests that, while sapovirus is reputed to cause benign disease in young children and immunocompetent patients, it may cause severe gastroenteritis in immunocompromised subjects and therefore should not be taken lightly. As polyvalent intravenous immunoglobulins have been proposed to help cure norovirus infection, we used them in an attempt to clear this sapovirus infection [102]. The optimal dose of IVIg is not formalized. Patte, et al. [102] used 1 g/kg IVIg three times a week, then once a week depending on the symptoms. The smaller dose we used could have slowed the clinical state. Our patient was maintained on intravenous immunoglobulins for a month as an anti-infectious prophylactic measure, as is common for stem cell transplant patients. The efficacy of this strategy for preventing secondary calicivirus infections remains to be determined.
In conclusion, sapovirus is a note-worthy emerging enteric virus that is similar to norovirus and may cause severe, prolonged diarrhoea in immunocompromised patients. Its potential for causing nosocomial epidemics remains to be clarified. It is diagnosed by RT-PCR analysis of fecal samples and its treatment is mainly symptomatic: intravenous or oral polyvalent immunoglobulins may be used as an experimental treatment to accelerate virus clearance, though the details of their efficacy remain to be studied.
- International Committee on Taxonomy of Viruses (ICTV) 2017. [Ref.]
- Reuter G, Zimsek-Mijovski J, Poljsak-Prijatelj M, Bartolo DI, Ruggeri FM, et al. (2010) Incidence, diversity, and molecular epidemiology of sapoviruses in swine across Europe. J Clin Microbiol 48: 363-368. [Ref.]
- Soma T, Nakagomi O, Nakagomi T, Mochizuki M (2015) Detection of Norovirus and Sapovirus from diarrheic dogs and cats in Japan. Microbiol Immunol 59: 123-128. [Ref.]
- Leblanc D, Inglis GD, Boras VF, Brassard J, Houde A (2017) The prevalence of enteric RNA viruses in stools from diarrheic and nondiarrheic people in southwestern Alberta, Canada. Arch Virol 162: 117-128. [Ref.]
- Lu Z, Yokoyama M, Chen N, Oka T, Jung K, et al. (2015) Mechanism of Cell Culture Adaptation of an Enteric Calicivirus, the Porcine Sapovirus Cowden Strain. J Virol 90: 1345-1358. [Ref.]
- Nakanishi K, Tatsumi M, Kinoshita-Numata K, Tsugawa T, Nakata S, et al. (2011) Full sequence analysis of the original Sapporo virus. Microbiol Immunol 55: 657-660. [Ref.]
- Oka T, Yamamoto M, Katayama K, Hansman GS, Ogawa S, et al. (2006) Identification of the cleavage sites of sapovirus open reading frame 1 polyprotein. J Gen Virol 87: 3329-3338. [Ref.]
- Yokoyama M, Oka T, Kojima H, Nagano T, Okabe T, et al. (2012) Structural basis for specific recognition of substrates by sapovirus protease. Front Microbiol 3: 312. [Ref.]
- Fullerton SW, Blaschke M, Coutard B, Gebhardt J, Gorbalenya A, et al. (2007) Structural and functional characterization of sapovirus RNA-dependent RNA polymerase. J Virol 81: 1858-1871. [Ref.]
- Hosmillo M, Sweeney TR, Chaudhry Y, Leen E, Curry S, et al. (2016) The RNA Helicase eIF4A Is Required for Sapovirus Translation. J Virol 90: 5200-5204. [Ref.]
- Hansman GS, Matsubara N, Oka T, Ogawa S, Natori K, et al. (2005) Deletion analysis of the sapovirus VP1 gene for the assembly of virus-like particles. Arch Virol 150: 2529-2538. [Ref.]
- Oka T, Yamamoto M, Miyashita K, Ogawa S, Katayama K, et al. (2009) Self-assembly of sapovirus recombinant virus-like particles from polyprotein in mammalian cells. Microbiol Immunol 53: 49-52. [Ref.]
- Oka T, Mori K, Iritani N, Harada S, Ueki Y, et al. (2012) Human sapovirus classification based on complete capsid nucleotide sequences. Arch Virol 157: 349-352. [Ref.]
- Hansman GS, Natori K, Oka T, Ogawa S, Tanaka K, et al. (2005) Crossreactivity among sapovirus recombinant capsid proteins. Arch Virol 150: 21-36. [Ref.]
- Hansman GS, Oka T, Sakon N, Takeda N (2007) Antigenic diversity of human sapoviruses. Emerg Infect Dis 13: 1519-1525.
- Oka T, Miyashita K, Katayama K, Wakita T, Takeda N (2009) Distinct genotype and antigenicity among genogroup II sapoviruses. Microbiol Immunol 53: 417-420. [Ref.]
- Chanit W, Thongprachum A, Khamrin P, Okitsu S, Mizuguchi M, et al. (2009) Intergenogroup recombinant sapovirus in Japan, 2007-2008. Emerg Infect Dis 15: 1084-1087. [Ref.]
- Dos Anjos K, Lima LM, Silva PA, Inoue-Nagata AK, Nagata T (2011) The possible molecular evolution of sapoviruses by inter- and intragenogroup recombination. Arch Virol 156: 1953-1959. [Ref.]
- Harada S, Oka T, Tokuoka E, Kiyota N, Nishimura K, et al. (2012) A confirmation of sapovirus re-infection gastroenteritis cases with different genogroups and genetic shifts in the evolving sapovirus genotypes, 2002-2011. Arch Virol 157: 1999-2003. [Ref.]
- Svraka S, Vennema H, van der Veer B, Hedlund KO, Thorhagen M, et al. (2010) Epidemiology and genotype analysis of emerging sapovirus-associated infections across Europe. J Clin Microbiol 48: 2191-2198. [Ref.]
- Iritani N, Yamamoto SP, Abe N, Kubo H, Oka T, et al. (2016) Epidemics of GI.2 sapovirus in gastroenteritis outbreaks during 2012-2013 in Osaka City, Japan. J Med Virol 88: 1187-1193. [Ref.]
- Lasure N, Gopalkrishna V (2017) Epidemiological profile and genetic diversity of sapoviruses (SaVs) identified in children suffering from acute gastroenteritis in Pune, Maharashtra, Western India, 2007- 2011. Epidemiol Infect 145: 106-114. [Ref.]
- Hansman GS, Ishida S, Yoshizumi S, Miyoshi M, Ikeda T, et al. (2007) Recombinant sapovirus gastroenteritis, Japan. Emerg Infect Dis 13: 786-788. [Ref.]
- Iizuka S, Oka T, Tabara K, Omura T, Katayama K, et al. (2010) Detection of sapoviruses and noroviruses in an outbreak of gastroenteritis linked genetically to shellfish. J Med Virol 82: 1247-1254. [Ref.]
- Fioretti JM, Rocha MS, Fumian TM, Ginuino A, da Silva TP, et al. (2016) Occurrence of human sapoviruses in wastewater and stool samples in Rio De Janeiro, Brazil. J Appl Microbiol 121: 855-862. [Ref.]
- Murray TY, Taylor MB (2015) Quantification and molecular characterisation of human sapoviruses in water sources impacted by highly polluted discharged wastewater in South Africa. J Water Health 13: 1055-1059. [Ref.]
- Grant LR, O’Brien KL, Weatherholtz RC, Reid R, Goklish N, et al. (2017) Norovirus and Sapovirus Epidemiology and Strain Characteristics among Navajo and Apache Infants. PLoS One 12: e0169491. [Ref.]
- Lorrot M, Bon F, El Hajje MJ, Aho S, Wolfer M, et al. (2011) Epidemiology and clinical features of gastroenteritis in hospitalised children: prospective survey during a 2-year period in a Parisian hospital, France. Eur J Clin Microbiol Infect Dis 30: 361-368. [Ref.]
- Thongprachum A, Khamrin P, Maneekarn N, Hayakawa S, Ushijima H (2016) Epidemiology of gastroenteritis viruses in Japan: Prevalence, seasonality, and outbreak. J Med Virol 88: 551-570. [Ref.]
- Nakamura N, Kobayashi S, Minagawa H, Matsushita T, Sugiura W, et al. (2016) Molecular epidemiology of enteric viruses in patients with acute gastroenteritis in Aichi prefecture, Japan, 2008/09-2013/14. J Med Virol 88: 1180-1186. [Ref.]
- Osborne CM, Montano AC, Robinson CC, Schultz-Cherry S, Dominguez SR (2015) Viral gastroenteritis in children in Colorado 2006-2009. J Med Virol 87: 931-939. [Ref.]
- Liu X, Jahuira H, Gilman RH, Alva A, Cabrera L, et al. (2016) Etiological Role and Repeated Infections of Sapovirus among Children Aged Less than 2 Years in a Cohort Study in a Peri-urban Community of Peru. J Clin Microbiol 54: 1598-1604. [Ref.]
- Mikula C, Springer B, Reichart S, Bierbacher K, Lichtenschopf A, et al. (2010) Sapovirus in adults in rehabilitation center, upper Austria. Emerg Infect Dis 16: 1186-1187. [Ref.]
- Lee LE, Cebelinski EA, Fuller C, Keene WE, Smith K, et al. (2012) Sapovirus outbreaks in long-term care facilities, Oregon and Minnesota, USA, 2002-2009. Emerg Infect Dis 18: 873-876.
- Hassan-Ríos E, Torres P, Muñoz E, Matos C, Hall AJ, et al. (2013) Sapovirus gastroenteritis in preschool center, Puerto Rico, 2011. Emerg Infect Dis 19: 174-175. [Ref.]
- Torner N, Martinez A, Broner S, Moreno A, Camps N, et al. (2016) Epidemiology of Acute Gastroenteritis Outbreaks Caused by Human Calicivirus (Norovirus and Sapovirus) in Catalonia: A Two Year Prospective Study, 2010- 2011. PLoS One 11: e0152503. [Ref.]
- Chhabra P, Payne DC, Szilagyi PG, Edwards KM, Staat MA, et al. (2013) Etiology of viral gastroenteritis in children <5 years of age in the United States, 2008-2009. J Infect Dis 208: 790-800. [Ref.]
- Chhabra P, Samoilovich E, Yermalovich M, Chernyshova L, Gheorghita S, et al. (2014) Viral gastroenteritis in rotavirus negative hospitalized children <5 years of age from the independent states of the former Soviet Union. Infect Genet Evol 28: 283-288. [Ref.]
- Kobayashi S, Fujiwara N, Yasui Y, Yamashita T, Hiramatsu R, et al. (2012) A food borne outbreak of sapovirus linked to catered box lunches in Japan. Arch Virol 157: 1995-1997. [Ref.]
- Brown JR, Shah D, Breuer J (2016) Viral gastrointestinal infections and norovirus genotypes in a paediatric UK hospital, 2014-2015. J Clin Virol 84: 1-6. [Ref.]
- Nakagawa-Okamoto R, Arita-Nishida T, Toda S, Kato H, Iwata H, et al. (2009) Detection of multiple sapovirus genotypes and genogroups in oyster-associated outbreaks. Jpn J Infect Dis 62: 63-66. [Ref.]
- Shirato-Horikoshi H, Ogawa S, Wakita T, Takeda N, Hansman GS (2007) Binding activity of norovirus and sapovirus to histo-blood group antigens. Arch Virol 152: 457-461. [Ref.]
- Bucardo F, Carlsson B, Nordgren J, Larson G, Blandon P, et al. (2012) Susceptibility of children to sapovirus infections, Nicaragua, 2005- 2006. Emerg Infect Dis 18: 1875-1878. [Ref.]
- Cubitt WD, McSwiggan DA, Arstall S (1980) An outbreak of calicivirus infection in a mother and baby unit. J Clin Pathol 33: 1095-1098. [Ref.]
- Farkas T, Deng X, Ruiz-Palacios G, Morrow A, Jiang X (2006) Development of an enzyme immunoassay for detection of sapovirusspecific antibodies and its application in a study of seroprevalence in children. J Clin Microbiol 44: 3674-3679. [Ref.]
- Hosmillo M, Sorgeloos F, Hiraide R, Lu J, Goodfellow I, et al. (2015) Porcine sapovirus replication is restricted by the type I interferon response in cell culture. J Gen Virol 96: 74-84. [Ref.]
- Lee RM, Lessler J, Lee RA, Rudolph KE, Reich NG, et al. (2013) Incubation periods of viral gastroenteritis: a systematic review. BMC Infect Dis 13: 446. [Ref.]
- Lee YW, Yang SI, Kim JM, Kim JY (2013) Clinical features and role of viral isolates from stool samples of intussuception in children. Pediatr Gastroenterol Hepatol Nutr 16: 162-170. [Ref.]
- Model L, Burnweit CA (2016) Sapovirus Gastroenteritis in Young Children Presenting as Distal Small Bowel Obstruction: A Report of 2 Cases and Literature Review. Case Rep Surg 2016. [Ref.]
- Yoshida T, Kasuo S, Azegami Y, Uchiyama Y, Satsumabayashi K, et al. (2009) Characterization of sapoviruses detected in gastroenteritis outbreaks and identification of asymptomatic adults with high viral load. J Clin Virol 45: 67-71. [Ref.]
- Sakai Y, Nakata S, Honma S, Tatsumi M, Numata-Kinoshita K, et al. (2001) Clinical severity of Norwalk virus and Sapporo virus gastroenteritis in children in Hokkaido, Japan. Pediatr Infect Dis J 20: 849-853. [Ref.]
- Iwakiri A, Ganmyo H, Yamamoto S, Otao K, Mikasa M, et al. (2009) Quantitative analysis of fecal sapovirus shedding: identification of nucleotide substitutions in the capsid protein during prolonged excretion. Arch Virol 154: 689-693. [Ref.]
- Rockx B, De Wit M, Vennema H, Vinjé J, De Bruin E, et al. (2002) Natural history of human calicivirus infection: a prospective cohort study. Clin Infect Dis 35: 246-253. [Ref.]
- Roos-Weil D, Ambert-Balay K, Lanternier F, Mamzer-Bruneel MF, Nochy D, et al. (2011) Impact of norovirus/sapovirus-related diarrhea in renal transplant recipients hospitalized for diarrhea. Transplantation 92: 61-69. [Ref.]
- Moser O, Lück S, Dilloo D, Eis-Hübinger AM, Simon A (2011) Sapovirus as a gastrointestinal pathogen in febrile pediatric patients with cancer. J Med Virol 83: 2233-2236. [Ref.]
- Oka T, Wang Q, Katayama K, Saif LJ (2015) Comprehensive Review of Human Sapoviruses. Clin Microbiol Rev 28: 32-53. [Ref.]
- Hansman GS, Guntapong R, Pongsuwanna Y, Natori K, Katayama K, et al. (2006) Development of an antigen ELISA to detect sapovirus in clinical stool specimens. Arch Virol 151: 551-561. [Ref.]
- Amin MR, Siddiqui MS, Ahmed D, Ahmed F, Hossain A (2011) Band T-cell epitope mapping of human sapovirus capsid protein: an immunomics approach. Int J Bioinforma Res Appl 7: 287-298. [Ref.]
- Kitamoto N, Oka T, Katayama K, Li TC, Takeda N, et al. (2012) Novel monoclonal antibodies broadly reactive to human recombinant sapovirus-like particles. Microbiol Immunol 56: 760-770. [Ref.]
- Chen Z, Sosnovtsev SV, Bok K, Parra GI, Makiya M, et al. (2013) Development of Norwalk virus-specific monoclonal antibodies with therapeutic potential for the treatment of Norwalk virus gastroenteritis. J Virol 87: 9547-9557. [Ref.]
- Liu J, Kibiki G, Maro V, Maro A, Kumburu H, et al. (2011) Multiplex reverse transcription PCR Luminex assay for detection and quantitation of viral agents of gastroenteritis. J Clin Virol 50: 308-313. [Ref.]
- Wolffs PFG, Bruggeman CA, van Well GTJ, van Loo IHM (2011) Replacing traditional diagnostics of fecal viral pathogens by a comprehensive panel of real-time PCRs. J Clin Microbiol 49: 1926- 1931. [Ref.]
- Jiang Y, Fang L, Shi X, Zhang H, Li Y, et al. (2014) Simultaneous detection of five enteric viruses associated with gastroenteritis by use of a PCR assay: a single real-time multiplex reaction and its clinical application. J Clin Microbiol 52: 1266-1268. [Ref.]
- Coudray-Meunier C, Fraisse A, Martin-Latil S, Delannoy S, Fach P, et al. (2016) A Novel High-Throughput Method for Molecular Detection of Human Pathogenic Viruses Using a Nanofluidic RealTime PCR System. PloS One 11: e0147832. [Ref.]
- Thongprachum A, Khamrin P, Pham NT, Takanashi S, Okitsu S, et al. (2017) Multiplex RT-PCR for rapid detection of viruses commonly causing diarrhea in pediatric patients. J Med Virol 89: 818-824.
- Hergens MP, Nederby Öhd J, Alm E, Askling HH, Helgesson S, et al. (2017) Investigation of a food-borne outbreak of gastroenteritis in a school canteen revealed a variant of sapovirus genogroup V not detected by standard PCR, Sollentuna, Sweden, 2016. Euro Surveill 22: 30543.
- Khare R, Espy MJ, Cebelinski E, Boxrud D, Sloan LM, et al. (2014) Comparative evaluation of two commercial multiplex panels for detection of gastrointestinal pathogens by use of clinical stool specimens. J Clin Microbiol 52: 3667-3673. [Ref.]
- Zhang C, Niu P, Hong Y, Wang J, Zhang J, et al. (2015) A probe-free four-tube real-time PCR assay for simultaneous detection of twelve enteric viruses and bacteria. J Microbiol Methods 118: 93-98. [Ref.]
- Buss SN, Leber A, Chapin K, Fey PD, Bankowski MJ, et al. (2015) Multicenter evaluation of the BioFire FilmArray gastrointestinal panel for etiologic diagnosis of infectious gastroenteritis. J Clin Microbiol 53: 915-925. [Ref.]
- Liesman RM, Binnicker MJ (2016) The role of multiplex molecular panels for the diagnosis of gastrointestinal infections in immunocompromised patients. Curr Opin Infect Dis 29: 359-365. [Ref.]
- Zhou Y, Wylie KM, El Feghaly RE, Mihindukulasuriya KA, Elward A, et al. (2016) Metagenomic Approach for Identification of the Pathogens Associated with Diarrhea in Stool Specimens. J Clin Microbiol 54: 368-375. [Ref.]
- HL Choi, Suh CI, Park SW, Jin JY, Cho HG, et al. (2015) Whole-Genome Sequencing Analysis of Sapovirus Detected in South Korea. PloS One 10: e0132328. [Ref.]
- de Graaf M, van Beek J, Koopmans MP (2016) Human norovirus transmission and evolution in a changing world. Nat Rev Microbiol 14: 421-433. [Ref.]
- Baldridge MT, Turula H, Wobus CE (2016) Norovirus Regulation by Host and Microbe. Trends Mol Med 22: 1047-1059. [Ref.]
- Belliot G, Lopman BA, Ambert-Balay K, Pothier P (2014) The burden of norovirus gastroenteritis: an important foodborne and healthcare-related infection. Clin Microbiol Infect 20: 724-730. [Ref.]
- Bucardo F, Reyes Y, Svensson L, Nordgren J (2014) Predominance of norovirus and sapovirus in Nicaragua after implementation of universal rotavirus vaccination. PloS One 9: e98201. [Ref.]
- Teunis PF, Moe CL, Liu P, Miller SE, Lindesmith L, et al. (2008) Norwalk virus: how infectious is it? J Med Virol 80: 1468-1476. [Ref.]
- Gastañaduy PA, Hall AJ, Curns AT, Parashar UD, Lopman BA (2013) Burden of norovirus gastroenteritis in the ambulatory setting-- United States, 2001-2009. J Infect Dis 207: 1058-1065. [Ref.]
- Hall AJ, Lopman BA, Payne DC, Patel MM, Paul A, et al. (2013) Norovirus disease in the United States. Emerg Infect Dis 19: 1198- 1205. [Ref.]
- Patel MM, Widdowson MA, Glass RI, Akazawa K, Vinjé J, et al. (2008) Systematic literature review of role of noroviruses in sporadic gastroenteritis. Emerg Infect Dis 14: 1224-1231. [Ref.]
- Verstraeten T, Jiang B, Weil JG, Lin JH (2016) Modelling Estimates of Norovirus Disease in Patients with Chronic Medical Conditions. PloS One 11: e0158822. [Ref.]
- Lindesmith L, Moe C, Marionneau S, Ruvoen N, Jiang X, et al. (2003) Human susceptibility and resistance to Norwalk virus infection. Nat Med 9: 548-553. [Ref.]
- Green KY (2014) Norovirus infection in immunocompromised hosts. Clin Microbiol Infect 20: 717-723. [Ref.]
- Bok K, Green KY (2012) Norovirus Gastroenteritis in Immunocompromised Patients. N Engl J Med 367: 2126-2132. [Ref.]
- Frange P, Touzot F, Debré M, Héritier S, Leruez-Ville M, et al. (2012) Prevalence and clinical impact of norovirus fecal shedding in children with inherited immune deficiencies. J Infect Dis 206: 1269-1274. [Ref.]
- Wingfield T, Gallimore CI, Xerry J, Gray JJ, Klapper P, et al. (2010) Chronic norovirus infection in an HIV-positive patient with persistent diarrhoea: a novel cause. J Clin Virol 49: 219-222. [Ref.]
- Rodríguez-Guillén L, Vizzi E, Alcalá AC, Pujol FH, Liprandi F, et al. (2005) Calicivirus infection in human immunodeficiency virus seropositive children and adults. J Clin Virol 33: 104-109. [Ref.]
- Ghosh N, Malik FA, Daver RG, Vanichanan J, Okhuysen PC (2017) Viral associated diarrhea in immunocompromised and cancer patients at a large comprehensive cancer center: a 10-year retrospective study. Infect Dis 49: 113-119. [Ref.]
- Lee LY, Ladner DP, Ison MG (2016) Norovirus infection in solid organ transplant recipients: a single-center retrospective study. Transpl Infect Dis 18: 932-938. [Ref.]
- Schorn R, Höhne M, Meerbach A, Bossart W, Wüthrich RP, et al. (2010) Chronic norovirus infection after kidney transplantation: molecular evidence for immune-driven viral evolution. Clin Infect Dis 51: 307-314. [Ref.]
- van Beek J, van der Eijk AA, Fraaij PL, Caliskan K, Cransberg K, et al. (2017) Chronic norovirus infection among solid organ recipients in a tertiary care hospital, the Netherlands, 2006-2014. Clin Microbiol Infect 23: e9-e265. [Ref.]
- Roddie C, Paul JP, Benjamin R, Gallimore CI, Xerry J, et al. (2009) Allogeneic hematopoietic stem cell transplantation and norovirus gastroenteritis: a previously unrecognized cause of morbidity. Clin Infect Dis 49: 1061-1068. [Ref.]
- Lemes LGN, Corrêa TS, Fiaccadori FS, Cardoso Dd, Arantes Ade M, et al. (2014) Prospective study on Norovirus infection among allogeneic stem cell transplant recipients: prolonged viral excretion and viral RNA in the blood. J Clin Virol 61: 329-333. [Ref.]
- Schwartz S, Vergoulidou M, Schreier E, Loddenkemper C, Reinwald M, et al. (2011) Norovirus gastroenteritis causes severe and lethal complications after chemotherapy and hematopoietic stem cell transplantation. Blood 117: 5850-5856.
- Bull RA, Eden JS, Luciani F, McElroy K, Rawlinson WD, et al. (2012) Contribution of intra- and inter host dynamics to norovirus evolution. J Virol 86: 3219-3229. [Ref.]
- Vega E, Donaldson E, Huynh J, Barclay L, Lopman B, et al. (2014) RNA populations in immunocompromised patients as reservoirs for novel norovirus variants. J Virol 88: 14184-14196. [Ref.]
- Hasing ME, Hazes B, Lee BE, Preiksaitis JK, Pang XL (2016) A next generation sequencing-based method to study the intra-host genetic diversity of norovirus in patients with acute and chronic infection. BMC Genomics 17: 480. [Ref.]
- Bruggink LD, Hayes L, Marshall JA (2015) Chronic norovirus infection in a patient with a past history of Burkitt lymphoma. Virusdisease 26: 207-210. [Ref.]
- Doerflinger SY, Weichert S, Koromyslova A, Chan M, Schwerk C, et al. (2017) Human Norovirus Evolution in a Chronically Infected Host. mSphere 2: e00352-e00416. [Ref.]
- Saif MA, Bonney DK, Bigger B, Forsythe L, Williams N, et al. (2011) Chronic norovirus infection in pediatric hematopoietic stem cell transplant recipients: a cause of prolonged intestinal failure requiring intensive nutritional support. Pediatr Transplant 15: 505-509. [Ref.]
- Knoll BM, Lindesmith LC, Yount BL, Baric RS, Marty FM (2016) Resolution of diarrhea in an immunocompromised patient with chronic norovirus gastroenteritis correlates with constitution of specific antibody blockade titer. Infection 44: 551-554. [Ref.]
- Patte M, Canioni D, Fenoel VA, Frange P, Rabant M, et al. (2017) Severity and outcome of the norovirus infection in children after intestinal transplantation. Pediatr Transplant 21: 12930. [Ref.]
- Florescu DF, Hermsen ED, Kwon JY, Gumeel D, Grant WJ, et al. (2011) Is there a role for oral human immunoglobulin in the treatment for norovirus enteritis in immunocompromised patients? Pediatr Transplant 15: 718-721. [Ref.]
- Kim Y, Galasiti Kankanamalage AC, Chang KO, Groutas WC (2015) Recent Advances in the Discovery of Norovirus Therapeutics. J Med Chem 58: 9438-9450. [Ref.]
- Thorne L, Arias A, Goodfellow I (2016) Advances toward a Norovirus Antiviral: From Classical Inhibitors to Lethal Mutagenesis. J Infect Dis 213: S27-S31. [Ref.]
- Damalanka VC, Kim Y, Galasiti Kankanamalage AC, Lushington GH, Mehzabeen N, et al. (2017) Design, synthesis, and evaluation of a novel series of macrocyclic inhibitors of norovirus 3CL protease. Eur J Med Chem 127: 41-61. [Ref.]
- Rocha-Pereira J, Van Dycke J, Neyts J (2016) Norovirus genetic diversity and evolution: implications for antiviral therapy. Curr Opin Virol 20: 92-98. [Ref.]
- SS Kaufman, KY Green, BE Korba (2014) Treatment of norovirus infections: moving antivirals from the bench to the bedside. Antiviral Res 105: 80-91. [Ref.]
- Ohba M, Oka T, Ando T, Arahata S, Ikegaya A, et al. (2017) Antiviral effect of theaflavins against caliciviruses. J Antibiot (Tokyo) 70: 443- 447. [Ref.]
- Koopmans M, von Bonsdorff CH, Vinjé J, de Medici D, Monroe S (2002) Foodborne viruses. FEMS Microbiol Rev 26: 187-205. [Ref.]
- Thebault A, Le Saux JC, Pommepuy M, Le Guyader S, Lailler R, et al. (2012) Quantitative approach of risk management strategies for hepatitis a virus-contaminated oyster production areas. J Food Prot 75: 1249-1257. [Ref.]
- Pavoni E, Consoli M, Suffredini E, Arcangeli G, Serracca L, et al. (2013) Noroviruses in seafood: a 9-year monitoring in Italy. Foodborne Pathog Dis 10: 533-539. [Ref.]
- Stals A, Van Coillie E, Uyttendaele M (2013) Viral genes everywhere: public health implications of PCR-based testing of foods. Curr Opin Virol 3: 69-73. [Ref.]
- Debbink K, Lindesmith LC, Baric RS (2014) The state of norovirus vaccines. Clin Infect Dis 58: 1746-1752. [Ref.]
- Aliabadi N, Lopman BA, Parashar UD, Hall AJ (2015) Progress toward norovirus vaccines: considerations for further development and implementation in potential target populations. Expert Rev Vaccines 14: 1241-1253. [Ref.]
Download Provisional PDF Here
Aritcle Type: CASE REPORT
Citation: Rousset S, Gauthier M, Huynh A, Mansuy JM (2018) Sapovirus-Associated Severe, Prolonged Diarrhoea in an Autologous Stem-Cell Transplant Patient: Case Report and Literature Review. J Emerg Dis Virol 4(2): dx.doi. org/10.16966/2473-1846.143
Copyright: © 2018 Rousset S, et al. This is an open-access article distributed under the terms of the Creative Commons Attribution License, which permits unrestricted use, distribution, and reproduction in any medium, provided the original author and source are credited.
Publication history:
SCI FORSCHEN JOURNALS
All Sci Forschen Journals are Open Access