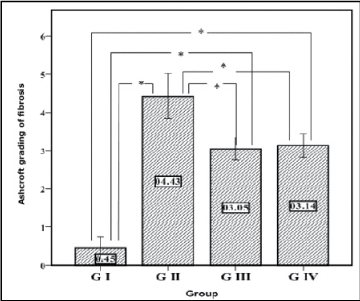
Figure 1: Descriptive data of Ashcroft grading of fibrosis results. Plotted values represent the arithmetic mean, and error bars represent the standard deviation. *means significant difference between groups (p<0.05).
Angie M Ameen1 Magda I Mohamed1 Mohammad Abdo1 Maha M Atwa2* Yasser M El-Wazir1
1Physiology Department, Faculty of Medicine, Suez Canal University, Ismaillia, Egypt*Corresponding author: Maha M Atwa, Department of Pathology, Faculty of Medicine, Suez Canal University, Ismailia, Egypt, Tel: 002-01223489703; Fax: 002-062-3192762; E-mail: mahaatwa99@gmail.com
Background: Pulmonary fibrosis is an interstitial lung disease characterized by progressive pulmonary parenchymal fibrosis which has no effective therapy available for it.
Aim of the work: The study aims assessing the ability of human umbilical cord blood CD34+ stem cells and mesenchymal stem cells (MSCs) to reduce pulmonary fibrosis in bleomycin rat model.
Materials and Methods: Thirty two rats divided into 4 equal groups were included in this study; group I: normal control group, group II: lung fibrosis group, group III: CD34+ group and group IV: MSCs group. Pulmonary fibrosis was induced in groups II, III and IV by bleomycin hydrochloride. On the day of induction, groups III and IV were treated by CD34+ stem cells and MSCs respectively. Fourteen days after induction of fibrosis, lung function was assessed by blood gas analysis, animals were sacrificed, and lungs were dissected for histopathology, immunohistochemistry and TGF-β1 assessment.
Results: Treatment with CD34+ stem cells significantly improved arterial blood oxygen. MSCs also improved blood oxygenation without restoring it to normal. Regarding degree of fibrosis, both CD34+ stem cells and mesenchymal stem cells were found to be markedly improved histopathological changes without restoring lung morphology to normal. Both stem cells restored TGF-β1 level to normal.
Conclusion: Treatment with CD34+ stem cells and MSCs improve functional and structural alterations in bleomycin-induced lung fibrosis in rats and CD34+ stem cells are better than MSCs in improving blood oxygenation.
Keywords: CD34+cells; Human umbilical cord blood; Mesenchymal stem cells; Pulmonary fibrosis; TGF-β1
Idiopathic pulmonary fibrosis is a cryptogenic lethal disease characterized by distortion of lung architecture accompanied by exaggerated matrix deposition resulting in loss of functional lung volume and gas exchanging capacity. Patients with late-stage pulmonary fibrosis (PF) mostly die of respiratory failure, the accompanying cardiac disease or lung infections [1].
In the last decade, major accomplishments have been achieved in decoding, diagnosing and treating PF as many new drugs and clinical guidelines have been advanced and utilized in PF patients. Clinical trials have tested a number of single or combination drug therapies to overwriting new clinical guidelines, but unfortunately the mortality of PF is still rising regardless of these advances [2]. There is general agreement that clinical trials should no longer be preventing further disease progression, but rather stabilizing lung function and exercise capacity [3].
Stem cell therapy has shed some new light on the management of PF as expert agreements have been achieved to favor stem cell therapy in chronic airway diseases and PF. The therapeutic role of mesenchymal stem cells (MSCs) has been implicated in serial processes of lung injuryrepair disequilibrium and abnormal remolding in experimental lung fibrosis. Clinical trials have been launched to investigate the clinical safety and efficacy of MSCs in PF patients [4].
Bleomycin is the most widely used drug for chemical induction of PF in animal models. It is highly reproducible and produces fibrotic histologic picture similar to the human disease. Bleomycin instillation in rat lungs causes PF through an inflammatory process characterized by patchy parenchymal inflammation of variable intensity, epithelial cell injury with reactive lymphoid hyperplasia, basement membrane damage, and interstitial as well as intra-alveolar fibrosis contributing the pathologic landmarks in both human PF and this model [5].
Some studies demonstrated the ability of adult tissue derived stem cells to differentiate into lung epithelial cells and confer a functional benefit [6]. This contribution of adult stem cells to the regeneration of lung tissue could be used as an alternative approach to the treatment of certain lung diseases. However, other studies failed to prove that adult stem cells could restore lung epithelium [7].
Concerning MSCs, Ortiz et al. [8] found that administration of these cells immediately after exposure to bleomycin rat significantly reduced the degree of bleomycin-induced inflammation and collagen deposition within lung tissue. Collectively, these studies demonstrated that murine MSCs home to the lung in response to injury, adopt an epithelium-like phenotype, and reduce inflammation and collagen deposition in lung tissue of mice challenged with bleomycin.
To the best of our knowledge, no previous study of stem cell therapy of induced PF has tested CD34+ stem cells. Given the regenerative capacity of CD34+ hematopoietic stem cells (HSCs) in hematological diseases, we decided to assess the reparative effect of this type of stem cells in murine model of induced PF structurally and functionally, and compare their results with those of MSCs.
A total of 32 adult female albino local strain rats, weighting 250–300 gm each, were used in this study. Animals were purchased from the National Research Centre, Cairo, Egypt. Animals were housed in polyethylene cages at room temperature (under controlled environmental conditions) and kept with free access to standard rodent chow diet and tap water ad libtium. Animals were allowed to acclimatize for one week before the start of the study. All efforts were made to minimize animal suffering and to reduce the number of animals used.
Rats were equally divided into four groups (8 rats each), group I (GI): the control untreated group included normal rats which did not receive any medications or stem cells, group II (GII) lung fibrosis group, group III (GIII) CD34+ stem cells treated group and group IV (GIV) MSCs treated group. PF was induced by bleomycin hydrochloride (Nippon Kayaku, Tokyo, Japan) in a dose of 1.5 mg/kg by intra-tracheal instillation as a single dose [9] in GII, GIII and GIV. On the day of induction GIII and GIV were treated with CD34+ cells and MSCs respectively in a dose of 1 × 106 cells/rat intravenously in the tail vein without immunosuppression.
The day of lung fibrosis induction was defined as day 0. Fourteen days (stage of fibrosis and collagen deposition). Later, arterial blood samples were withdrawn for blood gases analysis and then rats were sacrificed by cervical decapitation [10].
Human umbilical cord blood collection: Human umbilical cord blood (HUCB) samples were obtained from the umbilical cord while the placenta was still in utero in sterile collection tubes (50 ml) containing citrate phosphate dextrose adenine-1 (CPDA-1) as anticoagulant (5 ml/ tube). HUCB samples were obtained from healthy full-term neonates. Each sample was stored at 4°C and processed within 24 hours [11,12].
Isolation of HUCB mononuclear cells (MNCs): MNCs were isolated by Ficoll-Hypaque density gradient centrifugation method [13]. Cord blood was diluted 1:1 with phosphate buffered saline (PBS, LonzaBioproducts, Belgium) and carefully loaded onto histopaque-1077 solution (Sigma-Aldrich, USA). After density gradient centrifugation 2600 rpm for 35 min at room temperature, MNCs were removed from the interphase and washed many times with PBS.
Separation and purification of CD34+ stem cells: CD34+ cells were separated from MNCs by immunomagnetic separation technique [14] (Dynalbeads Oslo Norway) according to the manufacturer’s instructions. In the first step, CD34+ cells were captured by the Dynabeads M-450 CD34 and isolated using magnetic particle concentrator (Dynal MPC). In the second step, beads were removed from the cells by incubation with DETACHaBEAD CD34.
Selection of CD105+ CD34- CD45- MSCs: MSCs were isolated by using MACS technique where these cells lack the expression of CD34 and CD45 while express CD105 [15]. CD45– cells were separated using CD45 Microbeads Kits (Milteny Biotec, #130-045-801) and CD105+ cells were separated using CD105 Micro beads Kits (MiltenyiBiotec, #130-051-201). After CD34+ cells separation, CD34− fraction was washed with PBS and resuspended in MACS buffer and incubated with CD45 micro Beads, then washed and proceed to magnetic separation and the unlabeled cell fraction CD45– was taken [16]. The cell fractions of CD34− CD45– were washed and the cell pellet was resuspended in MACS buffer and incubated with CD105 micro Beads. The cells were washed and then proceed to magnetic separation and the labeled cell fraction CD105+ was taken [17]. The cell fraction obtained was finally CD34− CD45– CD105+.
Culture of MSCs: The CD34− CD45– CD105+ MSCs cultures were initiated in culture flasks of 25 cm2 (Greiner Bio-One, Germany). Cells were nurtured with Alpha Minimum Essential Medium (á-MEM, Lonza) supplemented with 20% fetal bovine serum (FBS, South American origin, Lonza), 1% antibiotic/antimycotic (Lonza) and 1% glutamine (Lonza). Cultures were incubated at 37°C, humidified atmosphere containing 5% CO2 . After overnight incubation, non adherent cells were removed and fresh medium was added to the culture flask. Later on, the culture medium was changed at every four days and cellular growth assessed daily under an inverted microscope. When the cells reached 50-60% confluence, they were harvested after trypsinization with trypsin/EDTA solution (ethylene-diamine-tetra-acetic acid, 0.025%) [18].
Functional assessment of the lung: Rats were anesthetized and blood was drained from the abdominal aorta into a heparinized syringe and immediately used for analysis. Blood analysis was performed with blood gas analyzer. For each rat, arterial pH, arterial oxygen and carbon dioxide tensions (PO2 and PCO2 ) and arterial bicarbonate (HCO- 3 ) concentration was measured [19].
Preparation of lung sections for histopathologicand immunohistochemical studies: After sacrifice, the thorax of each rat was opened and the hilum of each lung was ligated. Lungs were dissected free from their bronchi, blood vessels and hilar nodes and then collected in ice-cold container. Lungs were perfused free of blood with ice-cold saline. Right lungs were fixed in 10% neutral buffered formalin for histopathologicand immunohistochemical examination.
Histopathological examination: Lung samples were fixed in 10% formalin buffer for histological examination and routinely processed. Sections 3–4 µm thick were cut, stained with Haematoxylin and Eosin (H&E) and Masson’s Trichrome for assessing fibrotic lesions and microscopic observation. For the quantitative histological analysis, a numerical fibrotic scale (Ashcroft score) was used for the fibrosis score, with grades from 0 to 8 in which grade 0: normal lung, grade 1: minimal fibrous thickening of alveolar or bronchiolar vessels, grade 2: mild fibrous thickening of alveolar septa with knot-like formation, group 3: moderate thickening of the walls without obvious damage to lung architecture, grade 4: single fibrotic masses, group 5: confluent fibrotic masses with damage of lung architecture, group 6: large contiguous fibrous masses, group 7: sever distortion of lung architecture with honeycomb pattern, and group 8: total fibrous obliteration of lung architecture [20,21].
Immunohistochemical assessment for actin expression: About 4 µm sections from the paraffin blocks were mounted on super frost slides. Sections were deparaffinized and rehydrated in graded alcohol using Heat Induced Epitope Retrival (HIER) techniques using Cell Marque’s Triology in conjunction with a pressure cooker followed by cooling at room temperature for 20 minutes. Sections were treated for 5 min with 1% hydrogen peroxide to block endogenous peroxidase activity. Sections were stained using anti-smooth muscle actin antibody (Dako, USA) and Avidin-Biotin Detection System, DAB ready to use, Cell Marque, USA. The slides were counterstained with hematoxylin and mounted.
Assessment of Transforming Growth Factor β1 (TGF-β1 ): Nonlavaged whole left lungs were excised and homogenized on ice in 2 mL of cold PBS. After centrifugation at 4°C (10,000 rpm, 15 min), supernatants were divided into several aliquots and stored at -70°C for determination of TGF-β1 . Concentration of TGF-β1 was quantified using ELISA kits (DRG international company) according to the manufacturer [22].
Statistical analysis: Results were expressed as mean ± SD; tabulated and proper statistical analysis was done using SPSS statistical package, the difference between groups was assessed by ANOVA and post-Hoc Tukey test. P-value of <0.05 was considered significant.
Blood gas analysis showed significant improvement of GIII (CD34+ treated group) as arterial blood oxygen of this group differed significantly from GII (p=0.000) but it did not differ significantly from GI (p=0.092 ). This means that CD34+ cells restored arterial blood oxygenation to normal. GIV (MSCs treated group) showed arterial blood oxygen which differed significantly from GII (p=0.006) and GI (p=0.000). This showed that MSCs improved blood oxygenation but it could not restore it to normal levels (Table 1).
Regarding PO2 results, ANOVA test showed that there is significant difference of PO2 findings among all groups (p=0.000). Post-hoc Tukey test revealed that there was significant difference between GII and the other three groups (I, III, IV) which means that the mean level of PO2 was significantly lower in GII (untreated) than GI (normal) (p=0.000), GIII (CD 34+ treated group) (p=0.000) and GIV (MSCs treated group) (p=0.0006). There was no significant difference between GIII and GIV (p=0.137) and between GI and GIII (p=0.092). However, GI differed significantly from GIV (p=0.000).
ANOVA test showed that there was no statistically significant difference between groups regarding PCO2 , HCO3- and pH findings among groups (p=0.405, 0.817 and 0.609) respectively.
Table 1 showed that the mean PO2 level was lowest in GII (66.6 ± 6.3 mmHg) and GI was the highest (90 ± 3.35 mmHg). The mean PCO2 level was the highest in GII (47.23 ± 11.36 mmHg) and GI has the lowest PCO2 level (40.265 ± 8.99 mmHg). The mean HCO3-level was lowest in GI (22.20 ± 3.67 mmol/L) and highest in GII (23.36 ± 2.47 mmol/L). The mean pH level in GII was the lowest (7.32 ± 0.08) among the four groups.
Concerning histopathological changes, Ashcroft grading of fibrosis in GIII did not differ significantly from GIV (p=0.971). Also, both groups III and IV had a significantly lower grade than GII (p=0.000 and 0.000) respectively. These groups (III & IV) had a significantly higher grade than GI (p=0.000 and 0.000) respectively (Figure 1). Both types of stem cells were found to improve pathologic changes as compared with GII without complete restoration of lung morphology to normal.
Figure 1: Descriptive data of Ashcroft grading of fibrosis results. Plotted values represent the arithmetic mean, and error bars represent the standard deviation. *means significant difference between groups (p<0.05).
Figure 2 shows means and standard deviation of tissue levels of TGF- β1 . GII had the highest levels of TGF- β1 (179.54 ± 32.67 pg/ml) while GI had the lowest levels (139.08 ± 6.86 pg/ml). Post-hoc test revealed that, there was significant difference between GII and the other three groups (I, III, IV) which means that the mean level of TGF-β1 was significantly higher in GII (untreated) than GI (normal) (p=0.002), GIII (CD34+ treated group) (p=0.01) and GIV (MSCs treated group) (p=0.022). There was no significant difference between GIII and GIV (p=0.986) and between GI and GIII (p=0.905) and IV (p=0.741).
Figure 2: Descriptive data of TGF-β1 (pg/ml) results. Plotted values represent the arithmetic mean, and error bars represent the standard deviation. * means significant difference between groups (p<0.05).
Microscopic examination of the pulmonary tissue of the bleomycin challenged GII revealed severe inter-alveolar and intra-alveolar leukocytic infiltration with narrowing of alveolar spaces (Figure 4B) leading to marked thickening of interalveolar septa along with increased lymphoid follicle aggregates (Figure 3B) as compared with normal appearance of parenchyma (Figure 3A) and interalveolar septa of the control normal group (GI) (Figure 4A). Moderate inter-alveolar and intra-alveolar leukocytic infiltration (Figure 4D) along with moderate thickening of the inter-alveolar spaces was noticed in the MSCs treated group (GIV) with patchy areas restoring normal alveolar architecture (Figure 3D) while better histologic changes was noticed in CD34 +ve treated group (GIII) that shows mild thickening of the inter-alveolar spaces with more patchy areas restoring normal alveolar architecture (Figure 3C). There is evidence of mild leukocytic infiltration (Figure 4C).
Figure 3: Haematoxylin and Eosin staining of pulmonary tissue (X100). A: Pulmonary tissue of normal group (GI), shows normal appearance of parenchyma and interalveolar septa (H&E X100). B: Pulmonary tissue of bleomycin-challenged group (GII) shows thickening of interalveolar septa with narrowing of alveolar spaces and the presence of some lymphoid follicle aggregations (H&E, X100). C: Pulmonary tissue of GIII (CD34+ treated group) shows mild thickening of inter-alveolar septa along with patchy areas of normal tissue (H&E, X100). D: Pulmonary tissue of GIV (MSCs treated group) shows moderate thickening of interalveolar septa (H&E, X100).
Figure 4: Haematoxylin and Eosin staining of pulmonary tissue (X400). A: Pulmonary tissue of GI shows normal appearance of parenchyma and interalveolar septa (H&E, X400). B: Pulmonary tissue of GII shows thickening of interalveolar septa due to severe inter-alveolar and intraalveolar leukocytic infiltration with collapse of alveolar spaces (H&E, X400). C: Pulmonary tissue of GIII shows mild inter-alveolar leukocytic infiltration with mild thickening of inter-alveolar septa (H&E, X400). D: Pulmonary tissue of GIV shows moderate inter-alveolar and intraalveolar leukocytic infiltration with moderate thickening of inter-alveolar septa (H&E, X400).
The degree of fibrosis and the density of the collagen deposition were better demonstrated in Masson’s trichrome stained slides; GIII showed mild collagen deposition around alveolar walls (Figure 5C) and also GIV showed mild collagen deposition around alveolar wall and around blood vessels (Figure 5D) as compared with dense deposition of collagen and collapse of the alveolar spaces in GII (Figure 5B) and the normal appearance of alveoli in GI (Figure 5A).
Figure 5: Masson’s trichromestaining of pulmonary tissue (X400). A: Pulmonary tissue of GI shows normal appearance of parenchyma and interalveolar septa (Masson’s trichrome, X400). B: Pulmonary tissue of GII shows collapse of alveolar spaces and dense deposition of collagen around the alveolar walls (Masson’s trichrome, X400).C: Pulmonary tissue of GIII shows mild deposition of collagen around the alveolar walls (Masson’s trichrome, X400). D: Pulmonary tissue of GIV shows mild deposition of collagen around the alveolar walls and around blood vessels (Masson’s trichrome, X400).
Examination of the immunostained slides for Actin highlighted the early collagen deposition around the alveolar spaces in GIII (Figure 6C) and GIV (Figure 6D) while less collagen deposition around the alveolar spaces was detected in GIV but more actin positive cells was detected around the blood vessels in this group as compared with thick fibrous bands in GII (Figure 6B) and the normal appearance of GI (Figure 6A).
Figure 6: Anti-smooth muscle actin immunostaining of pulmonary tissue (X100). A: Pulmonary tissue of GI shows normal appearance of parenchyma and interalveolar septa with positive internal control of the blood vessels and smooch muscle fibers in adjacent tissue (Actin & DAB X100). B: Pulmonary tissue of GII shows fibrous bands formation between alveolar spaces (arrows) and dense deposition of collagen around the alveolar walls (Actin & DAB X100). C: Pulmonary tissue of GIII shows patchy deposition of collagen around the alveolar walls (arrows) (Actin & DAB X100). D: Pulmonary tissue of GIV shows less deposition of patchy collagen (relative to GIII) around the alveolar walls (arrows) (Actin & DAB X100)
The present study addresses the antifibrotic effect of stem cells derived from umbilical cord blood (UCB) against bleomycin induced PF in rats. Stem cells obtained from cord blood (CB), appear to have the capacity to develop into non-hematopoeitic cell types. In vitro studies have demonstrated the ability of CB-MSCs and CB-CD34+ stem cells to differentiate into non-hematopoietic tissues of all germ layers under specific culture conditions [23]. In vivo, CB-MSCs and CB-CD34+ stem cells have been studied as an alternative treatment approach for neurologic, liver, and cardiac diseases [24]. The first studied type in our work was the MSCs that supposed to have an effective role in treatment of PF in previous studies [6,8]. The other type was CD34+ stem cells which is the hematopoietic stem cell. We compared between the effect of CD34+ stem cells and MSCs both structurally by studying lung morphology and functionally by arterial blood gas analysis on bleomycin induced PF in rats.
Blood gas analysis showed significant improvement of CD34+ group with restoration of arterial blood oxygenation to normal. On the other hand, MSCs improved blood oxygenation but could not restore it to normal levels. The effect of CD34+ stem cells on blood oxygenation can be related to the marker that these cells carry (CD34). Mohty et al. [25] revealed that, the most commonly used surrogate marker for hematopoietic stem cells (HPCs) is the cell surface marker CD34 which is identified in the clinical laboratory by flow cytometry. Suratt et al. [26] demonstrated that bone marrow derived HSCs reformed 42% of lung blood vessels versus only 8% of lung epithelium. This finding supports our point of view that HSCs has the ability to reform blood vessels more than MSCs do. Consequently, they ameliorated gaseous exchange in a better way. Finally, Yoder and Ingram [27] demonstrated the presence of a subset of endothelial progenitor cells (EPCs) in culture. These cells exhibit cobblestone morphology that is a characteristic of mature endothelial cells. These EPCs express the endothelial markers as CD34. However they do not express monocytic markers. These cells have the ability to form vessels in vitro and in vivo (in lung and heart models). HSCs used in our study were CD34+, this might be the reason of these cells ability to preserve endothelial function in lung vasculature through maintaining gaseous exchange. This might be the reason that MSCs did not ameliorate arterial blood oxygen as significantly as CD34+ cells.
Ashcroft grading of lung tissue showed marked improvement in lung fibrosis of the two treated groups with no significant statistical correlation could be detected between them. In this study we found noticeable improvement in PF through the use of both CD34+ stem cells and mesenchymal ones; however CD34+ stem cells was found to be more favorable in treatment practice because they are much easier to obtain. Concerning MSCs, early cultures grow slowly and are heavily contaminated by hematopoietic cells that usually require immunoselection to remove [28].
Administration of CD34+ stem cells or MSCs immediately after bleomycin challenge protects lung tissue from bleomycin induced injury, as evidenced by a significant reduction in inflammation along with decreased leukocytic infiltration and collagen deposition evidenced by histopathology, and grading of fibrosis. Our study showed patchy collagen deposition in CD34+ stem cells GIII and in MSCs-GIV characterized by smooth muscle actin expression, as compared with the bleomycin-treated rats GII as smooth muscle actin positive cells represent the cell type most responsible for the increase in lung type collagen deposition indicating that these cells appear to play an important role in the pathogenesis of PF, and their presence may contribute to the increased extracellular matrix deposition and contractility of lung tissue in PF.
These findings indicate that systemic administration of CB derived MSCs and CD34+ stem cells may be beneficial in the treatment of PF. Furthermore, in our experimental approach, we suggest that MSCs obtained by both plastic adherence and immunoselection (CD34- , CD45- , CD105+), provide a direct measure of their contribution (not contaminated by hematopoietic stem cells) to the outcome. MSCs obtained only through plastic adherent cultures contain a variety of hematopoietic cell types, which persist in the cultures even after serial passage and exhibit an appreciable engraftment potential in vivo [29]. We found that MSCs ameliorated lung fibrosis grade and this was consistent with many studies that used MSCs. MSCs were hypothesized to limit the injurious effects of bleomycin by replacing alveolar epithelial type II cells. These cells are thought to function as stem cells in lung and are known targets of apoptotic signals induced in lung by bleomycin [30]. Restoration of the stem cell pool may partially occur through differentiation of MSCs into epithelial type II cells. This leads to increased genesis of alveolar cells which helps in the resolution of disrupted alveolar surfaces and augmenting the repair process [31,32].
Concerning CD34+ stem cells, Suratt et al. [26] demonstrated marked improvement of lung injury in response to this type of cells. These findings were consistent with the results of our study and in contrast with the findings of Wagers et al. [33]. In this study we selected a certain subpopulation of HSCs with different immunoselection markers. We used the most primary surrogate marker of HSCs which is CD34, assuming that we studied the effect of a broad range of CB derived HSCs.
We also studied the effect of both types of stem cells on TGF-β1 which is known to be a potent factor in the development of idiopathic PF [34] and animal experimental models of lung fibrosis [35,36]. It has been reported that the basis of the fibrogenic process is fibroblast proliferation and extracellular matrix accumulation [37]. We found that, the lung tissue level of TGF-β1 was significantly higher in bleomycin challenged group than the other three groups. We also found that the two types of stem cells returned the level of this cytokine to normal range as the level they achieved did not differ significantly from the normal group. There was also no significant difference between the two studied types of stem cells in their impact on the level of this cytokine although its level in CD34+ group was slightly lower than that obtained in the MSCs group. These results were consistent with Azuma et al. [38] who found that TGF-β1 level was increased in the rodent bleomycin-challenged animal lung fibrosis model. We suggest that the mechanisms by which stem cells ameliorate bleomycin-induced PF in rats may involve the inhibition of TGF-β1 signaling which is the main factor that mediates fibroblast proliferation and extracellular matrix production. Pathogenesis of idiopathic PF is suggested to begin with alveolitis which is characterized by the accumulation of inflammatory cells such as neutrophils and mononuclear cells. Concomitant cytokines like TGF-β1 are released to stimulate fibroblast proliferation. Fibroblasts then migrate into areas of acute lung injury and are stimulated to secrete collagen and other matrix proteins [39].
Ortiz et al. [40] concluded that MSCs may protect against bleomycin induced injury by altering the microenvironment of lung at sites of engraftment through the mechanism by which they may produce antagonists of TGF- β1 or other cytokines that disrupt signal pathways leading to fibrosis. Moreover, the study of Brody et al. [41] showed in vitro MSCs produce TGF- β1 .
Iso et al. [42] stated that umbilical cord blood derived MSCs (uMSCs) were detected in both fibrotic and non-fibrotic areas of the bleomycininjured lung at 14 days but they were not detected by 28 days after injection. Notably, uMSCs were not detected in the lungs of healthy mice at 14 to 28 days following injection, implying that tissue injury is necessary to attract and retain these cells. The repair of injured lung, despite the transient presence of uMSCs, is in consistence with previous reports involving other organs [43].
Our findings were consistent with the study of Ortiz et al. [40] and Baber et al. [44] that found uMSCs may ameliorate lung fibrosis through inhibition of the cytokine TGF-β1. This was demonstrated by the reduced fibrosis score on histology through reduction of collagen synthesis and deposition. Therefore, the regenerative influence of uMSCs may act through this important cytokine [40,44]. Bone marrow and CB derived MSCs were shown to be equally effective in their ability to express phenotypic markers of airway epithelium and to participate in airway remodeling in vivo [45]. Positive CD34 selection is also used in clinical HSC transplantations to deplete alloreactive T cells and possibly also solid tumor cells. This allows the collection and subsequent transplantation of mega doses of CD34+ cells, which can overcome histocompatibility barriers [46]. The advantages of CB for HSC transplantation include welldocumented lesser acute and chronic graft versus host disease when CB is used, compared to bone marrow [47].
In conclusion, CD34+ stem cells caused improvement of lung fibrosis both morphologically and functionally. This type moreover improved blood oxygenation better than MSCs. Blood oxygenation is the ultimate goal of any intervention in cases of lung injury. This effect obtained by HSCs is to be further assessed as it is a good way to improved quality of life in patients with lung diseases involving fibrotic processes.
Treatment with CD34+ stem cells and MSCs improved functional and structural alterations in bleomycin-induced lung fibrosis in rats and CD34+ stem cells were found to be better than MSCs in improving blood oxygenation.
There was no conflict of interest.
Download Provisional PDF Here
Aritcle Type: Research Article
Citation: Ameen AM, Mohamed MI, Abdo M, Atwa MM, El-Wazir YM (2016) Comparison between the Effect of CD34+ Stem Cells and Mesenchymal Stem Cells in Improving Pulmonary Fibrosis in Bleomycin Rat Model. Cell Stem Cells Regen Med 2(2): doi http://dx.doi.org/10.16966/2472-6990.112
Copyright: © 2016 Ameen AM, et al. This is an open-access article distributed under the terms of the Creative Commons Attribution License, which permits unrestricted use, distribution, and reproduction in any medium, provided the original author and source are credited.
Publication history:
All Sci Forschen Journals are Open Access