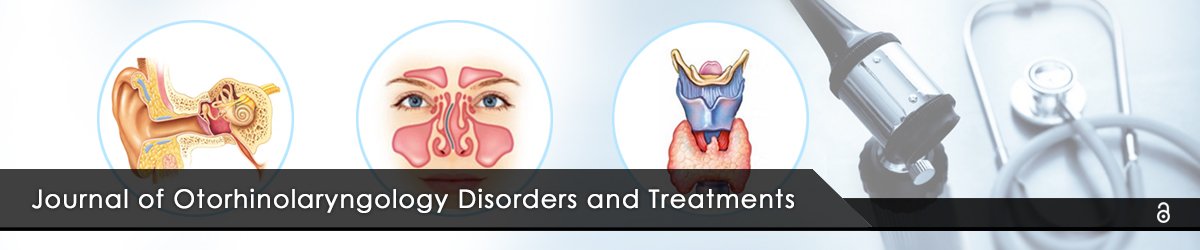
Full Text
Yunfang Zheng* Jianwei Guan
Department of Communication Sciences & Disorders, Central Michigan University, Mount Pleasant, MI 48859, United States*Corresponding author: Yunfang Zheng, Department of Communication Sciences & Disorders, Central Michigan University, Mount Pleasant, MI 48859, United States, Tel: 989-774-4405; E-mail: zheng4y@cmich.edu
Hearing loss is a common public health problem which will impact communication ability and hence hinge quality of life. Recent studies have found that instead of outer hair cells, the synapses between inner hair cells and auditory nerve fibers, especially low spontaneous rate nerve fibers, are most vulnerable to the effects of noise, aging, and ototoxic medication. The damage of synapses will cause sound-coding deficits in temporal and intensity processing leading to perceptual hearing difficulties, such as difficulty hearing in noise, tinnitus, hyperacusis, etc., but cannot be detected by current standard hearing diagnostic procedures. This pathology of synaptic loss in the cochlea was termed cochlear syanptopathy, more commonly called hidden hearing loss (HHL). This new finding inspired a line of studies leading to more detailed discoveries from underlying mechanisms to clinical applications, though they have not yet been widely recognized in the clinics. Currently, more people are struggling with HHL and it is occurring at a younger age due to increased recreational noise exposure. Due to a lack of awareness of synaptic dysfunction, no easily accessible diagnostic tools, and no effective treatments, people with HHL in the clinic are either informed of normal hearing sensitivity with no follow up services or referred for auditory processing disorder evaluation. This review provides intensive but condensed information about cochlear synaptopathy including etiology, diagnosis, differential diagnosis, and treatment, with the intention to guide professionals to a better understanding of this disorder, and hence leading to increased public awareness, early diagnosis, and prompt and proper intervention.
Cochlear synaptopaty; Hidden hearing loss; Auditory synaptopathy; Cochlear neuropathy
Recent research in mice by Kujawa and Liberman [1] found acute (within 24-hour post exposure) dramatic loss (up to 50%) of synapses between inner hair cells (IHCs) and afferent auditory nerve fibers (ANFs), post noise exposure with permanent amplitude reduction of auditory brainstem response (ABR) wave I and delayed (months post exposure) spiral ganglion loss. However, there was only a transient threshold shift and no hair cell loss, which led to the term cochlear synaptopathy, the pathology of synaptic loss in the cochlea. This challenged the previous assumption that outer hair cells are considered most vulnerable to noise, aging and drugs causing acquired sensorineural hearing loss, and inspired a subsequent line of studies. The above finding was confirmed by other animal studies [2-5]. It was also found that the IHC-ANF synapses were most vulnerable to aging [6,7] and dose-level-controlled ototoxic drugs [8]. Failing to accurately transmit auditory information to the brain due to cochlear synaptopathy, especially when the damage is selective to lowspontaneous-rate fibers due to drugs, noise exposure, and aging [9-11], will cause deficits in temporal and intensity coding [12], which will lead to common hearing loss associated symptoms, such as difficulty hearing in noise, tinnitus, and hyperacusis, etc. However, it will be hidden from standard clinical hearing diagnostic test (pure tone audiometry), which will show normal hearing sensitivity at 250-8000 Hz. Therefore, it was termed hidden hearing loss (HHL) by Schaette and McAlpine [13], and currently commonly used for this pathology. It is also called auditory synaptopathy [14] or cochlear neuropathy [10] due to the site of lesion and its neurological consequences.
HHL is common in humans, with the prevalence increasing with age [15], while currently it occurs at younger ages due to more noise exposure [16-18], and its perceptual difficulties due to deficits in temporal and intensity coding could be a major health issue [14]. Two studies that investigated the prevalence of HHL [15,19]) recruited 2783 (21-67 years old) and 2015 (20-69 years old) adults, respectively. The results indicated that 10.4 ~ 12% subjects reported hearing difficulties among those with normal hearing sensitivity at 250-8000 Hz, which is about 2.9 ~ 6.07% of the entire participated subjects. However, note that the reported hearing difficulties could be due to not just cochlear synaptopathy, but also other pathologies such as reduced cochlear function due to partial damage to OHCs [20], OHC damage up to 20-30% would not elevate the hearing thresholds), and central auditory and/or non-auditory deficits. Another study [21] conducted in a large audiology clinic facility found that about 5% of patients (<60 years old), including both children and adults, had self-reported hearing difficulties but with normal hearing sensitivity. The prevalence could be underestimated because unlike people with diagnosed or with more significant hearing loss, people with normal hearing sensitivity would be less likely referred or seek treatment. People with perceptual difficulty but with normal hearing thresholds would usually be formally informed that he or she has normal hearing and be provided with some communication strategies for improvement, or be referred for auditory processing disorder evaluation, especially for children with reported difficulties in school, due to a lack of awareness of synaptic dysfunction, easily accessible diagnostic tools, and effective treatments.
It’s important to have a better understanding of HHL to increase public awareness to prevent further possible auditory damage, and for professionals to possess the knowledge to have early diagnosis of this disorder and provide proper intervention, hence reduce possible health issues leading to a better quality of life. This article reviewed up to date studies related to cochlear synaptopathy including etiology, diagnosis, differential diagnosis, and treatment, with the intention to provide intensive but condensed information about cochlear synaptopathy to guide professionals through a better understanding of this disorder.
To understand cochlear synaptopathy, it is important to comprehend the anatomy and physiology of hair cells, synapses between the IHCs and ANFs, ANFs themselves, and neurons/ spiral ganglia (SG).
The organ of corti, a sensory organ of hearing in the cochlea, has two types of sensory hair cells: IHCs and outer hair cells (OHCs). The hair cells connect to ANFs through synapses, and each ANF has a cell called spiral ganglion [22]. The synaptic ribbon in IHCs is attached by synaptic vesicles containing neurotransmitters [23]. Normally, about 95% of the afferent nerve fibers, type I fiber, connect to IHCs only, and each bipolar SG only receives input from one IHC [24]. Also, each IHC has redundant synaptic innervation, about 5-30:1 ANF-IHC innervation ratio depending on different species [25], which leads to some resistance of functional deficit caused by synaptic loss.
The type I fiber can be further divided into three subgroups according to their spontaneous firing rate (SR). The high-SR fibers with low neuron thresholds have thicker axons and more mitochondria and are located on the pillar side of IHCs; the low SR fibers with the highest neuron thresholds have the thinnest axon and fewer mitochondria and are located on the modiolar side of IHCs; the mid-SR fibers are also on the modiolar side with characteristics between the other two fibers [26].
OHCs amplify the movement of the basilar membrane due to electromotility contributing to better hearing sensitivity to lowlevel sounds and improved frequency selectivity [27]. The role of IHCs is to transduce the mechanical energy from the middle ear to the electrical signal transmitted through the auditory nervous system. When sounds reach the ears, the hydromechanical energy pushes the basilar membrane upward, causing the stereocilia of the hair cells to move away from the modiolus of the cochlea, which initiates a depolarization process of the hair cells. In the IHCs a less negative depolarization potential generated by potassium influx triggers calcium influx from the presynaptic active zone of the synaptic ribbon, leading to a release of neurotransmitter (glutamate) from the synaptic vesicles to the synaptic cleft, which will then be picked up by the nerve endings and stimulates the SG to generate action potential/electrical impulses transferred to the brain [28]. The synaptic transmission has high temporal precision ensuring neural response phase-locked to the sound waveform [29,30]. Also, the combined responses from different nerve fibers or SGs ensure a wide dynamic range of intensity coding of acoustic sounds [31].
Faithfully transducing acoustic signals through the auditory system relies on an intact anatomy, physiology, and strong communication between auditory structures. Synaptic losses between IHCs and ANFs cause sound coding deficits in temporal and intensity processing, leading to different perceptual hearing difficulties [32]. Recently, most of the research studies have been focusing on acquired cochlear synaptopathy/HHL under the influence of noise, aging, and ototoxic medication.
Hair cells, especially OHCs, were historically considered most vulnerable to noise exposure, aging, and ototoxic drugs and will be damaged first, and neurons die due to hair cell degeneration [33-35], though it was found that partial OHC loss (up to 20 ~ 30% loss) will not elevate hearing thresholds [20]. However, recent studies have different findings.
Study on mice found acute (within 24hr) IHC-ANF synaptic loss (50%) occurring post noise exposure (100 dB SPL octave band noise for 2 hours) with temporal threshold shift (20- 40 dB) and delayed (months to years post exposure) spiral ganglion loss, while no loss of hair cells (both OHC & IHC) was found with normal stereocilia bundles up to at least one year post exposure [1]. Another study examined mice with no noise exposure longitudinally from 4-week (young) to 144- week (old). Synaptic loss was found with aging before threshold elevation and hair cell loss, and nerve loss was found severalmonth post synaptic loss [36]. Other animal studies confirmed cochlear synaptopathy without loss of hair cells due to aging and noise exposure [3,6,10]. A study on human post-mortem temporal bones (age 54-89 years) using immunostaining approaches also found cochlear synaptopathy with nearly intact hair cells [7]. In regard to the effects of ototoxic medication, a study by Ruan et al [8] using mice treated with different doses of gentamicin found that low-dose gentamicin caused more type I afferent nerve fiber dendrite loss than hair cell loss, and dendrite loss was independent from hair cell loss. The above findings suggest that synapses will be damaged first instead of hair cells due to noise exposure, aging, and ototoxic drugs. However, compared to rodents, larger animals may need higher noise levels and longer exposure duration to induce cochlear pathology and the pathology may not be limited to synapses. Valero et al [37] found that monkeys exposed to narrowband noise at 120 dB (one was exposed to 120) SPL for four hours had 12 ~ 27% synaptic loss at basal cochlea with scattered OHC loss and temporary threshold shift of 20 dB.
It was found that early noise exposure will exacerbate hearing loss with aging in humans [38] and in mice [39]. A longitudinal study [38] found that people with more previous noise exposure history had significantly more hearing threshold change compared to those with less noise exposure as they aged. However, the noise-age interaction will only happen when there is synaptopathy due to noise exposure. A study on mice found that noise exposure (100dB SPL) with even one acute synaptopathy exacerbated age related auditory change causing more synaptic loss and spiral ganglion loss, and threshold elevation spread to low frequency; there was no acceleration of the aging process in the cochlea when a transient threshold shift (TTS) was caused by a lower level exposure (91 dB) with no acute synaptopathy [40]. A 94 dB SPL was found the highest noise level causing TTS without causing synaptopathy on mice [2]. Studies found that rodents are more noise susceptible than humans, suggesting higher noise levels are needed to cause cochlear pathology for humans [41]. The noise level for rodent synaptopathy exceeds the safe daily exposure level for humans [42,43]. More studies on larger animals are needed to establish the highest level that will cause TTS but no synaptopathy, which will give more insight to human pathology due to noise exposure and possible combined effects of noise and age on hearing sensitivity.
Glutamate excitotoxicity (over production of neurotransmitters causing nerve ending swelling) in the IHC area was observed within 24 hours of noise exposure which initiated further neural degeneration [1]. This selective IHCdendrite excitotoxicicty was also reported in other studies post nose exposure [44,45], with aging [7], and ototoxic medication [46,47]. It was found that low-SR fibers were more vulnerable to noise exposure, aging, and drugs, and would be damaged foremost compared to other ANF subgroups [6,9,26,40,48]. This is supported by the damage observed in the modiolar side of the IHC and the fact that no elevation of the hearing threshold but permanent suprathreshold neural response reduction was indicated in the ABR measures. High-SR fibers, sensitive to low-level sounds contributing to threshold detection, will be saturated at the level low-SR fibers start responding to sounds [49]. Unlike the high-SR fibers, the lowSR fibers are the thinnest type I subgroup, located on the IHC modiolar side, with fewer mitochondria, and respond to high level sounds, which make them more susceptible/vulnerable to external effects.
Low-SR fibers have high thresholds and are insensitive to continuous noise, which may have specific contributions to hearing in noise [50]. In addition, Low-SR fibers were found important to the olivocochlear reflex [51] and acoustic reflex [52], which help protect hearing from loud noises by inhibiting the cochlear amplifier or increasing middle ear transduction impedance, respectively. Noise induced damage of low-SR fibers affecting the reflexes may cause further damage of the cochlea, leading to loss of hair cells and permanent threshold shift [5]. Also, both reflexes have more effect on low-frequency than high frequency sounds, which help signal detection in noise [53].
This selective damage of cochlear synaptopathy causes inaccurate intensity representation of acoustic signals and degraded temporal precision which are important for coding suprathreshold sounds, therefore leading to clinical functional deficits, such as difficulty hearing in noise, hyperacusis, tinnitus, etc. [12,32,54-57]. Hearing in noise is a difficult listening situation because the noise will mask the signal. The level of a tone signal needs to be increased to at least the same level as the noise to be detected [58]. When low-SR fibers are damaged, the ability to code/respond to high level sounds will be affected, especially in noisy environments, due to the following reasons. First, the intensity coding dynamic range will be reduced from the full range of intensity coding to a soft to moderate sound level coding due to losing high-level sound response from the low-SR fibers. Second, the intact high-SR fibers ensure normal hearing sensitivity in quiet but they will be easily masked by noise. Third, the improved hearing in noise ability is affected/ diminished because the olivocochlear reflex and acoustic reflex are affected due to low-SR damage. Fourth, degraded temporal precision and intensity coding caused by synaptopathy will cause inaccurate timing cues and intensity cues which are important for hearing in noise, to be transferred to the central auditory system, which further deteriorates the ability to detect signals in noise. Therefore, people with cochlear synaptopathy/ HHL will report difficulty hearing in noisy environments but still have normal hearing sensitivity in quiet.
Liberman et al [54] found young adults with high-risk noise exposure history had significantly more self-reported avoidance of loud sounds (hyperacusis) than low-risk young adults. The results of this experiment showed that this high-risk group had normal hearing thresholds (at 250-8000 Hz), elevated high frequency thresholds, poorer high frequency distortion product optoacoustic emission results, reduced suprathreshold neural response from ANFs (reduced amplitude of ABR wave I), and reduced hearing in noise performance. Selective damage of low-SR fibers leads to a reduced dynamic range of intensity coding, and affects the protective function from two reflexes (olivocochlear reflex and acoustic reflex) leading to vulnerability to loud sound exposure, which may attribute to hyperacusis.
Tinnitus is also one of the complaints that are considered associated with HHL. However, the relationship between tinnitus and HHL and the etiology of tinnitus remain unclear. Animal studies have showed that cochlear synaptopathy leads to tinnitus possibly due to compensatory central gain caused by impaired afferent neural input [13], which was further demonstrated by studies on human subjects.
It was found that tinnitus subjects had normal behavioral hearing thresholds and ABR thresholds with affected ANF responses (reduced ABR wave I amplitude, generated by combined auditory nerve activity) but unaffected central neural responses (normal wave V amplitude, generated by central neurons-lateral lemniscus) [13,59]. In addition, a study on military Veterans and non-Veterans with limited number of subjects found ABR wave I amplitude reduction had strong association with tinnitus but not hyperacusis [60]. However, some other studies did not find correlation between tinnitus and wave I amplitude reduction [61]. Also, a study on mice [62] found that cochlear neural degeneration caused by noise exposure was associated with enhanced startle responses (behavioral signs related to hyperacusis), but with limited gap detection deficits (associated with tinnitus). In the same study, the amplitude was reduced for ABR wave I but not wave V for mice with synaptic loss due to noise exposure. It was concluded that the impaired afferent neural input and compensatory central gain might lead to hyperacusis instead of tinnitus. Further studies are needed to investigate the association between tinnitus and HHL.
Studies have been focusing on synaptic loss as a contribution to HHL. Recently, it was found that transient loss of Schwann cells of the auditory nerve resulting in permanent loss of the first heminodes at the ANF is associated with HHL. This could be the reason causing auditory symptoms after recovering from acute demyelinating disorders such as Guillanin-Barre syndrome caused by the zika virus [63]. A study in mice showed that noise exposure inhibited the upregulation of Hepatocyte Nuclear Factor-4 alpha (HNF4α) due to aging, especially in the spiral ganglia. HNF4α suggestively could be important for spiral ganglion survival. Therefore, human disorders with HNF4α mutation and polymorphisms may exacerbate noise induced HHL [64]. Other studies found genetic mutation, such as mutation of OTOF and DFNA25 etc., will cause cochlear synaptopathy. However, the resulting hearing loss is more severe than HHL [14].
Pure tone audiometry is the current standard hearing diagnostic test, measuring the lowest detectable sound level at octave frequencies from 250 Hz to 8000 Hz. The results recorded in an audiogram indicate the degree, type, and configuration of hearing loss, diagnosed clinically when the threshold is more than 20dB HL (hearing level). However, the audiogram does not include all information of the hearing/auditory system and does not always reflect the reported perceptual difficulties [32]. In addition, the audiogram is relatively insensitive to neural dysfunction; the threshold may not be elevated until loss of up to 80-90% of IHCs, synapses, or auditory nerves [65,66]. Cochlear synaptopathy with about 50% synaptic loss is hidden from current audiological evaluations but demonstrates perceptual difficulties due to deficits in temporal and intensity coding [55,57,67]. Currently, there is no standard diagnostic procedure/battery available due to issues in test reliability and validation. Special immunostaining or serial-section electron microscopy needed for synaptic analysis to validate the diagnosis is not practical for human study [7]. High-field diffusion MRI with high resolution images may provide estimation of nerve loss [68]. However, the extent of nerve damage may not be detected by MRI right after the initial lesion because neurons may survive without the synapse for months or years [6]. A combined test battery including electrophysiological and behavioral measures would help reduce inter- or intra- subject variability shown in individual tests, reduce confounding factors contributed by central processing, and provide possible links between synaptopathy and perceptual difficulties [69,70].
The click-evoked ABR, a noninvasive electrophysiology measure, has been proven an effective diagnostic method for rodent cochlear synaptopathy. The amplitude of ABR wave I, indicating the combined auditory nerve response, is reduced permanently post noise exposure and with aging, and is only reduced for mid-high level stimuli but not for low level sounds, indicating the selective damage of low-SR fibers [13]. In addition, the amplitude was found to correspond to the amount of intact synapses post noise exposure [1] and with aging [36]. The consistency between the synaptic count and amplitude reduction validates the reliability of the wave I measure for diagnosing HHL for animals. Studies on human subjects found that ABR wave I is a noninvasive method for neural response but may not be reliable for individual HHL diagnosis [70]. Wave I amplitude was reduced due to noise exposure [71], aging [72], and for people with tinnitus [13], but has more inter- and intra-subject variability and relatively low amplitude due to differences in gender, anatomy, and physiology [48, 73-75]. It is suggested to ensure stable physical status across patients (relaxed/sleep) to reduce within- and between-subject variability. Some alternative diagnostic ABR measures were also suggested. Wave I/V ratio helps reduce within-subject variability, and could be a diagnostic method for cochlear synaptopathy. It’s found for tinnitus patients, wave I/V ratio was reduced due to the reduction of wave I amplitude but not wave V, which might be because of the central gain mechanism amplifying the central response (wave V amplitude not affected) to compensate for the reduced auditory nerve input [13]. Elevated Wave V/I ratio was also found in age related HHL in mice [36]. However, aging may cause amplitude reduction of both wave I and V due to neural degeneration [72]. In 2016, Mehraei et al. [55] investigated the effect of noise on ABR wave V latency in both humans and mice. The results indicated that the smaller masked wave V latency shift with increasing masking level corresponded to less wave I amplitude growth, and the wave V latency shift was consistent with the degree of cochlear synaptopathy demonstrated by synaptic ribbon count in mice. That is, the smaller the masked wave V latency shifts the more synaptic loss. This measurement was robust and with less inter-subject variability, and was hence suggested as a possible diagnostic method for cochlear synaptopathy in humans. However, wave V is primarily generated by the lateral lemniscus in the brainstem, which might be affected by central processing.
The summating potential/action potential (SP/AP) ratio recorded from electrocochleography measure was also suggested as a possible method for synaptopathy diagnosis. SP is a resting cochlear potential, and AP is the same as the ABR wave I indicating combined auditory nerve response. Using tiptrodes in electrocochleography recording will have a stronger or larger AP wave than recorded in ABR. Also, the SP/AP ratio limited inter-subject variability in electrophysiology measures caused by physical issues (e.g., internal noise, head size), electrode resistance, etc. Animal studies have found increased SP/AP ratio due to decreased AP associated to synaptopathy caused by noise exposure, aging, and drugs [1,36,76]. Young adults with noise exposure history also showed increased SP/ AP, but it’s because of both increased SP and decreased AP [54]. Increased SP could be due to increased cochlear response caused by impaired acoustic reflex and olivocochlear reflex because of loss of low-SR fibers.
Another auditory evoked potential, frequency or envelope following response (FFR or EFR), will phase lock to stimuli (both envelope and fine structure), and was suggested to be a diagnostic measure for cochlear synaptopathy. FFR measurement montage is similar to ABR, but with more robust amplitude. It was found that FFR amplitude was reduced in post-noise-exposure mice [48], listeners with noise exposure but normal hearing thresholds [12,48], and tinnitus subjects [77]. However, FFR may be affected by physiological noises like ABR. FFR amplitude would decrease with aging for normal hearing listeners due to both peripheral and central contributions because of its central origin (primarily from inferior colliculus in mid-brain) [78-80], and would be affected by individual central processing. Musicians and people who speak tonal languages had stronger FFRs possibly due to more experience in stimuli [81]. Therefore, FFR cannot be used individually for HHL diagnosis.
Some behavioral/psychoacoustic measures could be part of the test battery to identify perceptual difficulties associated with temporal and intensity coding deficits due to selective damage of low-SR nerve endings of cochlear synaptopathy. An animal study found reduced ABR wave I amplitude and decreased hearing in noise performance for a TTS post noise exposure [82]. It was also found that young adults with noise exposure history, normal threshold, and normal word recognition in quiet, had poorer recognition scores in noise, reverberation, and when speech stimuli is time-compressed [54], suggesting speech audiometry in difficult listening environments may assist the diagnosis of synaptopathy [83,84]. There are other studies targeting different perceptual difficulties. Elevated thresholds for intensity discrimination was found for tinnitus patients with normal hearing sensitivity but reduced ABR wave I [13,85]. Binaural detection thresholds were increased with increasing interaural time delay for listeners with hearing sensitivity above 7.5 dB HL but no greater than 25 dB HL, suggesting perceptual binaural processing difficulty even with normal clinical hearing thresholds [86]. Modulation detection was suggested to be a diagnostic test targeting temporal processing deficit [87]. Uncomfortable level (UCL) measure might be one of the diagnostic method to target hyperacusis. Reduced UCL was found for adolescents with noise exposure history and tinnitus compared to the control group [88]. However, psychoacoustic measures would be affected by individual central processing [70] due to the involvement of processing from the ascending auditory pathway to motor response (e.g., press the button or say the response), which includes not just peripheral auditory processing but also central auditory processing. Also, the results will be affected by memory and attention, the higher level processing functions which further causes individual differences. To reduce variability caused by learning for psychoacoustic tests, it was suggested to simplify the procedure and provide some practice to ensure the best performance of each subject [89].
Extended high-frequency (EHF) audiometry including testing frequencies above 8000 Hz is a simple tone-detection test with a short test time, and could be an effective screening tool for early diagnosis of HHL [90]. Animal studies indicated that basal cochlea hair cells were affected first due to noise exposure [26,91]. A study on young adults with noise exposure history showed normal hearing sensitivity at standard test frequencies but higher thresholds, above 8 kHz, compared to those in the low-risk group [54]. Another study on adults with reduced speech in noise performance but normal hearing thresholds at 500-8000 Hz showed elevated hearing thresholds at higher frequencies (above 8000 Hz) compared to control group [92]. Ototoxic medication, such as cisplatin, was also found to affect the basal cochlea first [93]. Compared to conventional pure tone audiometry, EHF audiometry was found more sensitive to the detection of the initial cochlear damage due to ototoxic medication [94,95]. EHF audiometry was recommended to be used clinically to monitor ototoxicity [96]. It was found that EHF audiometry and distortion product optoacoustic emission (DPOAE) measures had the same sensitivity for detecting cisplatin-induced ototoxicity [97]. DPOAE is a clinical objective measure for the function of OHCs. OHC dysfunction indicated in DPOAE was found in listeners with normal hearing sensitivity (10-25 dB HL) [98], and OHC function was correlated with the pure tone audiometric results and speech in noise performance [99]. Therefore, including DPOAE as part of the diagnostic protocol for HHL would not only indicate OHC function but also provide valuable information regarding underlying etiology of perceptual difficulty such as hearing difficulty in noise.
A recent human study on young adults with noise exposure and tinnitus found that tinnitus was related to lifetime noise exposure but not related to reduced ABR wave I amplitude and EFR measures [61]. Liberman et al. [54] found that normal hearing young adults with reported noise exposure history had HHL related perceptual difficulties and performed poorer in HHL related diagnostic evaluation tests than those with no reported noise exposure history. Both studies suggested the importance of case history on the diagnosis of HHL.
A study using wideband chirps found that mice with noise induced synaptopathy had elevated acoustic reflex thresholds and reduced reflex response amplitude, suggesting wideband acoustic reflex could be a diagnostic test for HHL [100]. Synaptic loss due to noise exposure with selective damage of low-SR fibers endings would cause abnormal acoustic reflex responses. Wideband stimuli were specially suggested to avoid further damage of the cochlea because they could reduce thresholds by 24dB compared to probe tone. However, there is no wideband acoustic reflex test available to humans.
In summary, more research is needed for establishing a standard diagnostic test/test battery, and a comprehensive test battery is necessary for diagnosis of HHL to reduce withinand between-subject variability and serve as a cross check to increase diagnostic accuracy. It should include a questionnaire targeting noise exposure history and hearing ability in different listening environments, a conventional comprehensive hearing evaluation (otoscopy, tympanometry, pure tone audiometry, speech audiometry in quiet, DPOAE) to provide basic hearing status and differentiate HHL from hearing loss, extended high frequency audiometry for early detection of HHL, behavioral tests in difficult environments (e.g., noise, time compression, reverberation) targeting perceptual difficulties, and electrophysiology measures (e.g., ABR wave I/V ratio, wave V latency, SP/AP ratio) to identify the neural based site of lesion.
Hearing difficulties with a normal audiogram can be caused by different issues. The pathology of HHL caused by cochlear synaptopathy is in the synaptic connection between IHC and ANF in the peripheral auditory system. HHL can also be a consequence of different central issues, such as auditory processing disorder (APD) due to deficits in the auditory brainstem or cortex, cognitive deficits, attention issues, or problems in working memory [64]. APD refers to a group of perceptual processing deficits that affect the central auditory modality [101]. A clinical diagnostic test battery including a group of psychoacoustic measures had good sensitivity and specificity for site of lesion [102]. However, temporal processing deficits associated with APD could also be a result of HHL due to peripheral lesions [103]. Electrophysiology evaluations such as ABR (response from auditory nerve to brainstem neurons), middle latency response (mid brain to subcortical neurons), and late evoked auditory potentials (central neurons), may help differentiate the site of lesion [101]. Other studies reported that poor performance in APD evaluation could be caused by cognitive deficits [104] and linguistic disorders [105]. It was recommended to have psychological or neurological assessments before APD evaluation to rule out other central modality deficits associated with attention, memory, etc., which also provided useful information for more controlled APD evaluation [64]. Recent complex ABR (cABR) using speech signals, more sensitive to subcortical speech processing, was found to have strong correlation with HINT scores [106,107], suggesting potential to differentiate HHL from auditory to cognitive contributions. The auditory continuous performance test could be used for attention issue [108]. Also, MRI might help confirm site of lesion [64].
People with auditory neuropathy (AN), can have a normal audiogram but with poorer than expected speech understanding ability, especially in noise. AN is a hearing disorder characterized by presenting otoacoustic emissions (OAEs) and/or cochlear microphonic (CM) with abnormal or absent ABR [14,109]. Click ABR would be sufficient to differentiate HHL from AN. Although AN could be caused by cochlear synaptopathy due to genetic mutations, such as the mutations of OTOF and DFNA25, the resulting hearing loss was more severe than HHL [14].
As stated in the Diagnosis session, the SP/AP ratio recorded from the electrocochleography measure was suggested as a possible method for synaptopathy diagnosis, showing increased SP/AP [54]. Increased SP/AP in electrocochleography was used for Meniere’s disease diagnosis [110], which could also be due to synaptopathy because significant loss of synapses was found for a unilateral Meniere’s disease [111]. Meniere’s disease usually has characteristic symptoms including fluctuating hearing loss (usually starts at low frequencies), vertigo, tinnitus (low pitch), and aural fullness, which would be helpful for differential diagnosis.
Studies have been trying to find treatments for HHL. Animal studies had demonstrated the effectiveness of using neurotrophin to treat cochlear synaptopathy. Neurotrophins, proteins from IHC area supporting cells, are important for ANF survival [112]. Genetically mediated over expression of neurotrophin-3 was found to help promote synaptic regeneration between IHCs and ANFs for mice post noise exposure and helped recovery of the ABR wave I amplitude [113]. Another study by Sly et al. [114] had similar outcomes. Guinea pigs were exposed to two levels of noises (95 dB or 105 dB SPL), which caused cochlear synaptopathy with no threshold elevation. Neurotrophin delivered to the round window immediately post noise exposure led to more synapses for both levels of exposure and recovery of neural function, indicated by recovery or improvement of ABR wave I amplitude for noise level at 95 dB and 105 dB SPL, respectively. The results were confirmed in a mice study [115]. However, similar trial has not been attempted using human subjects.
A study on mice delivered pharmacological inhibitor compound C via intraperitoneal injection for three scenarios (24-hour & 2-hour before, and immediately after exposure) and siRNA through intratympanic application to the round window (72-hour before noise exposure) with a result of a reduction of synaptic ribbon loss and OHC loss [116]. AMPactivated protein kinase (AMPK) plays an important role in cellular energy homeostasis and will be increased post noise exposure. Both compound C and siRNA will inhibit AMPK, attenuating cell and neural losses, and preserve auditory function, suggesting a possible prevention treatment to noise induced hearing loss [117]. However, no similar study has been conducted in humans.
Recent studies have been trying to demonstrate the effectiveness of using auditory training to improve perceptual hearing difficulties. Neural plasticity has been demonstrated in animal studies. A group of aged rats had an almost complete reversal of their functional deficits and degraded cortical representations post intensive auditory training via an “oddball discrimination task” [117]. A later study on human and mice by Whitton, Hancock, & Polley [118] used tone-in-noise tasks and found that both species had improved task performance with intensive training. In addition, human subjects had improved speech perception in noise post training, and the cortical neural response of mice changed to better decoding of the training sounds leading to improved hearing in noise. Other studies have demonstrated improved hearing in noise post training of listening tasks in noise for both animal and human subjects [105,119]. Music training was also found to improve auditory function even for people with synaptopathy [120]. In addition, low-level sound therapy was found helpful for symptoms with tinnitus and hyperacusis [121,122]. All of these proposed the possibility of using auditory training to reduce hearing deficits. Focusing on deficit specific tasks was suggested for maximum improvement [123].
Hearing aids, a proven effective rehabilitation method for individuals with mild to severe hearing loss, was demonstrated an effective treatment option for some individuals with hearing difficulties, such as speech in noise and tinnitus [124,125]. Roup et al. [124] recruited nineteen adults with normal hearing sensitivity but self-reported difficulty understanding speech in adverse listening environments, who were fitted with binaural mild-gain (5-10 dB gain at 1-4 kHz for soft and moderate inputs) receiver-in–the-canal hearing aids for four weeks. The post aided results indicated significant reduction of self-perceived hearing difficulties and clear improvement in speech-in-noise performance compared to unaided condition, suggesting the possibility of using binaural mild-gain amplification to treat some individuals with hearing difficulties, especially in noise. In 1985, Surr et al [125] found that about half of 124 hearing aid new users that experienced tinnitus reported symptom reduction with hearing aids, indicating the effectiveness of using hearing aids on tinnitus. The result was further confirmed by Del Bo and Ambrosetti [126], who reported about 60% hearing aid users had minor to major reduction of tinnitus. Hearing aids will help amplify sounds to ease communication to reduce personal stress, and the amplified external sounds will act as a masker to reduce the internal tinnitus. Besides, increased auditory stimulation may help reduce the recognition of internal soft tinnitus. All of these help the effectiveness of using hearing aids on tinnitus. Currently tinnitus program is included in different types of hearing aids with multiple treatment sounds, and hearing aids have been used clinically as one of the treatment methods for the tinnitus.
Since most HHLs are acquired, not congenital, the prevention of damage is important and possible. A sound level of 85 or 90 dB A (A-weighted SPL) is considered a safe daily exposure (8-hour) without causing permanent threshold shift, and the amount of exposure time should be reduced half with an additional 3 or 5 dB A level increase, according to the National Institute for Occupational Safety and Health (NIOSH) and the Occupational Safety and Health Administration (OSHA), respectively [42-43]. However, a study on mice found that exposure to lower level sounds (75 dB SPL 10-week) caused significant synaptopathy [64]. Lower level sound exposure (broadband noise at 76 dBA 8-hour, 4 kHz band noise at 65 dBA 4-hour) also caused mild transient threshold shift for humans [127]. In addition, even being exposed to just one impulse noise or having one blast injury could damage IHC-ANF synapses and/or hair cells [128]. Synaptopathy would induce delayed neural damages causing perceptual difficulties and exacerbate neural degeneration caused by aging [1,36]. It is important to protect hearing by wearing ear protection whenever exposed to loud sounds, and monitor hearing sensitivity for elders and people administered with ototoxic medications. It is necessary for those working in noisy environments to monitor hearing sensitivity regularly for early diagnosis and prompt intervention.
In summary, cochlear synaptopathy is a newly discovered disorder with damage occurring at synapses between IHCs and ANFs, specifically at low-SR nerve endings, causing perceptual difficulties such as difficulty hearing in noise, tinnitus, hyperacusis, etc., although it cannot be detected by current standard hearing diagnostic procedures. Therefore, it is commonly termed HHL. Multiple reasons could cause cochlear synaptopathy including noise exposure, age, ototoxic medication, viral infection, genetic mutation, etc. Although there are effective diagnostic methods for animals, currently no standard clinical diagnostic test battery is available for humans due to inter- and intra-variability and lack of practical validation methods. A comprehensive test battery is suggested to cross check and reduce the variability, including a comprehensive case history (targeting noise exposure history, HHL related perceptual difficulties, and some other possible causes), behavioral/psychoacoustic measures with difficult listening environments (targeting perceptual difficulties), electrophysiology measures to identify neural based site of lesion, an extended pure tone audiometry for early lesion detection, and current standard hearing evaluation procedures to provide basic hearing status and differentiate HHL from hearing loss. Differential diagnosis may include AN and Meniere’s disease, but more often central HHL which is caused by auditory modality (e.g., APD) and higher level cortical processing deficits (e.g., cognitive, attention, language deficits). Neurotrophin had been demonstrated an effective method to regenerate synapses and help recover neural function for animals. Pharmacological inhibitor compound C and siRNA could help prevent noise induced hearing loss for mice. However, no effective treatment is available for humans, although auditory training and hearing aids were found effective for improving perceptual deficits associated with HHL. Also, prevention is important and possible for acquired HHL. It is suggested to wear ear protection whenever exposed to loud noises to prevent damage to the auditory system, regularly monitor hearing sensitivity for elders, those working daily in noisy environments, and those who took or are taking ototoxic medication, for early diagnosis of hearing loss and to receive appropriate intervention for better quality of life.
HHL is currently becoming more common and occurring at younger ages due to increased recreational noise exposure, and its associated perceptual difficulties may cause major health issues. Audiologists should play a leading role in regard to the diagnosis, intervention, and education of HHL to increase public awareness, prevent potential auditory damage, and improve professional understanding of this disorder for early diagnosis. More studies are needed to investigate the prevalence and effective treatment of this disorder, establish diagnostic norms, and determine a diagnostic protocol that can be used universally and reliably
- Kujawa SG, Liberman MC (2009) Adding insult to injury: cochlear nerve degeneration after “temporary” noise-induced hearing loss. J Neurosci 29: 14077-14085. [Ref.]
- Jensen JB, Lysaght AC, Liberman MC, Qvortrup K, Stankovic KM (2015) Immediate and delayed cochlear neuropathy after noise exposure in pubescent mice. PLoS One 10: e0125160. [Ref.]
- Lin HW, Furman AC, Kujawa SG, Liberman MC (2011) Primary neural degeneration in the Guinea pig cochlea after reversible noise-induced threshold shift. J Assoc Res Otolaryngol 12: 605- 616. [Ref.]
- Niwa K, Mizutari K, Matsui T, Kurioka T, Matsunobu T, et al. (2016) Pathophysiology of the inner ear after blast injury caused by laser-induced shock wave. Sci Rep 6: 31754. [Ref.]
- Wang Y, Ren C (2012) Effects of repeated “benign” noise exposures in young CBA mice: shedding light on age-related hearing loss. J Assoc Res Otolaryngol 13: 505-515. [Ref.]
- Sergeyenko Y, Lall K, Liberman MC, Kujawa SG (2013) Age-related cochlear synaptopathy: an early-onset contributor to auditory functional decline. J Neurosci 33: 13686-13694. [Ref.]
- Viana LM, O’Malley JT, Burgess BJ, Jones DD, Oliveira CA, et al. (2015) Cochlear neuropathy in human presbycusis: Confocal analysis of hidden hearing loss in post-mortem tissue. Hear Res 327: 78-88. [Ref.]
- Ruan Q, Ao H, He J, Chen Z, Yu Z, et al. (2014) Topographic and quantitative evaluation of gentamicin-induced damage to peripheral innervation of mouse cochleae. Neurotoxicology 40: 86-96. [Ref.]
- Bourien J, Tang Y, Batrel C, Huet A, Lenoir M, et al. (2014) Contribution of auditory nerve fibers to compound action potential of the auditory nerve. J Neurophysiol 112: 1025-1039. [Ref.]
- Furman AC, Kujawa SG, Liberman MC (2013) Noise-induced cochlear neuropathy is selective for givers with low spontaneous rates. J Neurophysiol 110: 577-586. [Ref.]
- Schmiedt RA, Mills JH, Boettcher FA (1996) Age-related loss of activity of auditory-nerve fibers. J Neurophysiol 76: 2799-2803. [Ref.]
- Plack CJ, Barker D2, Prendergast G (2014) Perceptual consequences of “hidden” hearing loss. Trends Hear 18. [Ref.]
- Schaette R, McAlpine D (2011) Tinnitus with a normal audiogram: physiological evidence for hidden hearing loss and computational model. J Neurosci 31: 13452-13457. [Ref.]
- Moser T, Starr A (2016) Auditory neuropathy-neural and synaptic mechanisms. Nat Rev Neurol 12: 135-149. [Ref.]
- Spankovich C, Gonzalez VB, Su D, Bishop CE (2017) Self reported hearing difficulty, tinnitus, and normal audiometric thresholds, the National Health and Nutrition Examination Survey 1999- 2002. Hear Res 358: 30-36. [Ref.]
- Carter L, Williams W, Black D, Bundy A (2014) The leisure-noise dilemma: Hearing loss or hearsay? What does the literature tell us? Ear Hear 35: 491-505. [Ref.]
- Keith SE, Michaud DS, Feder K, Haider I, Marro L, et al. (2011) MP3 player listening sound pressure levels among 10 to 17 year old students. J Acoust Soci Am 130: 2756. [Ref.]
- Liberman MC (2015) Hidden hearing loss. Sci Am 313: 48-53. [Ref.]
- Tremblay KL, Pinto A, Fischer ME, Klein BE, Klein R, et al. (2015) Self-Reported Hearing Difficulties Among Adults With Normal Audiograms: The Beaver Dam Offspring Study. Ear Hear 36: e290-e299. [Ref.]
- Bohne BA, Harding GW (1992) Neural regeneration in the noisedamaged chinchilla cochlea. Laryngoscope 102: 693-703. [Ref.]
- Hind SE, Haines-Bazrafshan R, Benton CL, Brassington W, Towle B, et al. (2011) Prevalence of clinical referrals having hearing thresholds within normal limits. Int J Audiol 50: 708-716. [Ref.]
- Musiek FE, Baran JA (2007) The auditory system: Anatomy, physiology, and clinical correlates. Boston: Allyn and Bacon. [Ref.]
- Matthews G, Fuchs P (2010) The diverse roles of ribbon synapses in sensory neurotransmission. Nat Rev Neurosci 11: 812-822. [Ref.]
- Spoendlin H (1972) Innervation densities of the cochlea. Acta Otolaryngol 73: 235-248. [Ref.]
- Liberman MC, Dodds LW, Pierce S (1990) Afferent and efferent innervation of the cat cochlea: Quantitative analysis with light and electron microscopy. J Comp Neurol 301: 443-460. [Ref.]
- Liberman MC, Kiang NY (1978) Acoustic trauma in cats. Cochlear pathology and auditory-nerve activity. Acta Otolaryngol Suppl 358: 1-63. [Ref.]
- Ashmore J (2008) Cochlear outer hair cell motility. Physiol Rev 88: 173-210. [Ref.]
- Bear MF, Connors BW, Paradiso MA (2006) The Auditory and Vestibular Systems. Neuroscience: Exploring the brain. Baltimore, MD: Lippincott Williams & Wilkins. [Ref.]
- Fuchs PA (2005) Time and intensity coding at the hair cell’s ribbon synapse. J Physiol 566: 7-12. [Ref.]
- Moser T, Neef A, Khimich D (2006) Mechanisms underlying the temporal precision of sound coding at the inner hair cell ribbon synapse. J Physiol 576: 55-62. [Ref.]
- Palmer AR, Evans EF (1982) Intensity coding in the auditory periphery of the cat: Responses of cochlear nerve and cochlear nucleus neurons to signals in the presence of band-stop masking noise. Hear Res 7: 305-323. [Ref.]
- Bharadwaj HM, Masud S, Mehraei G, Verhulst S, ShinnCunningham BG (2015) Individual differnces reveal correlates of hidden hearing deficits. J Neurosci 35: 2161-2172. [Ref.]
- Bohne BA, Harding GW (2000) Degeneration in the cochlea after noise damage: primaryversus secondary events. Am J Otol 21: 505-509. [Ref.]
- Johnsson LG (1974) Sequence of degeneration of Corti’s organ and its first-order neurons. Ann Otol Rhinol Laryngol 83: 294-303. [Ref.]
- Johnsson LG, Hawkins JE Jr (1976) Degeneration patterns in human ears exposed to noise. Ann Otol Rhinol Laryngol 85: 725- 739. [Ref.]
- Sergeyenko Y, Lall K, Liberman MC, Kujawa SG (2013) Age-related cochlear synaptopathy: an early-onset contributor to auditory functional decline. J Neurosci 33: 13686-13694. [Ref.]
- Valero MD, Burton JA, Hauser SN, Hackett TA, Ramachandran R, et al. (2017) Noise-induced cochlear synaptopathy in rhesus monkeys (Macaca mulatta). Hear Res 353: 213-223.
- Gates GA, Schmid P, Kujawa SG, Nam B, D’Agostino R (2000) Longitudinal threshold changes in older men with audiometric notches. Hear Res 141: 220-228. [Ref.]
- Kujawa SG, Liberman MC (2006) Acceleration of age-related hearing loss by early noise exposure: evidence of a misspent youth. J Neurosci 26: 2115-2123. [Ref.]
- Fernandez KA, Jeffers PW, Lall K, Liberman MC, Kujawa SG (2015) Aging after noise exposure: acceleration of cochlear synaptopathy in “recovered” ears. J Neurosci 35: 7509-7520. [Ref.]
- Dobie RA, Humes LE (2017) Commentary on the regulatory implications of noise-induced cochlear neuropathy. Int J Audiol 56: 74-78. [Ref.]
- National Institute for Occupational Safety and Health (1998) Criteria for a recommended standard: Occupational noise exposure. [Ref.]
- Occupational Safety and Health Administration (2002) Hearing conservation.
- Spoendlin H (1971) Primary structural changes in the organ of Corti after acoustic overstimulation. Acta Otolaryngol 71: 166- 176. [Ref.]
- Puel JL, Ruel J, Gervais d’Aldin C, Pujol R (1998) Excitotoxicity and repair of cochlear synapses after noise-trauma induced hearing loss. Neuroreport 9: 2109-2114. [Ref.]
- Basile AS, Huang JM, Xie C, Webster D, Berlin C, et al. (1996) N-methyl-D-aspartate antagonists limit aminoglycoside antibiotic-induced hearing loss. Nat Med 2: 1338-1343. [Ref.]
- Duan M, Agerman K, Ernfors P, Canlon B (2000) Complementary roles of neurotrophin 3 and N-methyl-D-aspartate antagonist in the protection of noise and aminoglycoside-induced ototoxicity. Proc Natl Acad Sci U S A 97: 7597-7602. [Ref.]
- Shaheen LA, Valero MD, Liberman MC (2015) Towards a Diagnosis of Cochlear Neuropathy with Envelope Following Responses. J Assoc Res Otolaryngol 16: 727-745. [Ref.]
- Winter IM, Robertson D, Yates GK (1990) Diversity of characteristic frequency rate-intensity functions in Guinea pig auditory nerve fibers. Hear Res 45: 191-202. [Ref.]
- Costalupes JA, Young ED, Gibson DJ (1984) Effects of continuous noise back-grounds on rate response of auditory nerve fibers in cat. J Neurophysiol 51: 1326-1344. [Ref.]
- Liberman MC (1988) Physiology of cochlear efferent and afferent neurons: direct comparisons in the same animal. Hear Res 34: 179-191. [Ref.]
- Liberman MC, Kiang NY (1984) Single-neuron labeling and chronic pathology. IV. Stereocilia damage and alterations in rate and phase level functions. Hear Res 16: 75-90. [Ref.]
- Kawase T, Delgutte B, Liberman MC (1993) Antimasking effects of the olivocochlear reflex. II. Enhancement of auditory-nerve response to masked tones. J Neurophysiol 70: 2533-2549. [Ref.]
- Liberman MC, Epstein MJ, Cleveland SS, Wang H, Maison SF (2016) Toward a Differential Diagnosis of Hidden Hearing Loss in Humans. PLoS One 11: E0162726. [Ref.]
- Mehraei G, Hickox AE, Bharadwaj HM, Goldberg H, Verhulst S, et al. (2016) Auditory Brainstem Response Latency in Noise as a Marker of Cochlear Synaptopathy. J Neurosci 36:3755-3764. [Ref.]
- Shi L, Chang Y, Li X, Aiken SJ, Liu L, et al. (2016) Coding deficits in noise-induced hidden hearing loss may stem from incomplete repair of ribbon synapses in the cochlea. Front Neurosci 10: 231. [Ref.]
- Song Q, Shen P, Li X, Shi L, Liu L, et al. (2016) Coding deficits in hidden hearing loss induced by noise: the nature and impacts. Sci Report 6: 25200. [Ref.]
- Fletcher H (1940) Auditory patterns. Rev Mod Phys 12: 47. [Ref.]
- Xiong H, Chen L, Yang H, Li X, Qiu Z (2013) Hidden hearing loss in tinnitus patients with normal audiograms: implications for the origin of tinnitus. Lin Chung Er Bi Yan Hou Tou Jing Wai Ke Za Zhi 27: 362-365. [Ref.]
- Bramhall NF, Konrad-Martin D, McMillan GP (2018) Tinnitus and Auditory Perception After a History of Noise Exposure: Relationship to Auditory Brainstem Response Measures. Ear Hear. [Ref.]
- Guest H., Munro K.J, Prendergast G, Howe S, Plack CJ (2017) Tinnitus with a normal audiogram: Relation to noise exposure but no evidence for cochlear synaptopathy. Hear Res 344: 265- 274. [Ref.]
- Hickox AE, Liberman MC (2014) Is noise-induced cochlear neuropathy key to the generation of hyperacusis or tinnitus? J Neurophysiol 111: 552-564. [Ref.]
- Wan G, Corfas G (2017) Transient auditory nerve demyelination as a new mechanism for hidden hearing loss. Nat Commun 8: 14487. [Ref.]
- Groth JB, Kao SY, Briët MC, Stankovic KM (2016) Hepatocyte nuclear factor-4 alpha in noise-induced cochlear neuropathy. Dev Neurobiol 76: 1374-1386. [Ref.]
- Lobarinas E, Salvi R, Ding D (2013) Insensitivity of the audiogram to carboplatin induced inner hair cell loss in chinchillas. Hear Res 302: 113-120. [Ref.]
- Schuknecht HF, Woellner RC (1955) An experimental and clinical study of deafness from lesions of the cochlear nerve. J Laryngol Otol 69: 75-97. [Ref.]
- Oxenham AJ (2016) Predicting the Perceptual Consequences of Hidden Hearing Loss. Trends Hear. [Ref.]
- Song SK, Sun SW, Ju WK, Lin SJ, Cross AH (2003) Diffusion tensor imaging detects and differentiates axon and myelin degeneration in mouse optic nerve after retinal ischemia. NeuroImage 10: 1714-1722. [Ref.]
- Kobel M, Le Prell CG, Liu J, Hawks JW, Bao J (2017) Noise-induced cochlear synaptopathy: Past findings and future studies. Hear Res 349: 148-154. [Ref.]
- Plack CJ, Léger A, Prendergast G, Kluk K, Guest H (2016) Toward a Diagnostic Test for Hidden Hearing Loss. Trends Hear 20. [Ref.]
- Stamper GC, Johnson TA (2015) Auditory function in normalhearing, noise-exposed human ears. Ear Hear 36: 172-184. [Ref.]
- Konrad-Martin D, Dille M.F, McMillan G, Griest S, McDermott D, et al. (2012) Age-related changes in the auditory brainstem response. J Am Acad Audiol 23: 18-35. [Ref.]
- Beattie RC (1988) Interaction of click polarity, stimulus level, and repetition rate on the auditory brainstem response. Scand Audiol 17: 99-109. [Ref.]
- Don M, Ponton CW, Eggermont JJ, Masuda A (1994) Auditory brainstem response (ABR) peak amplitude variability reflects individual differences in cochlear response times. J Acoust Soc Am 96: 3476-3491. [Ref.]
- Mitchell C, Phillips DS, Trune DR (1989) Variables affecting the auditory brainstem response: Audiogram, age, gender, and head size. Hear Res 40: 75-86. [Ref.]
- Woellner RC, Schuknecht HF (1955) Hearing loss from lesions of the cochlear nerve: an experimental and clinical study. Trans Am Acad Ophthalmol Otolaryngol 59: 147-149. [Ref.]
- Paul BT, Bruce IC, Roberts LE (2017) Evidence that hidden hearing loss underlies amplitude modulation encoding deficits in individuals with and without tinnitus. Hear Res 344: 170-182. [Ref.]
- Bones O, Plack CJ (2015) Losing the music: Aging affects the perception and subcortical neural representation of musical harmony. J Neurosci 35: 4071-4080. [Ref.]
- Frisina RD, Walton JP (2006) Age-related structural and functional changes in the cochlear nucleus. Hear Res 216-217: 216-223. [Ref.]
- Krishnan A (2006) Frequency-following response. In RF Burkhard, M Don, J Eggermont (Eds) Auditory evoked potentials: Basic principles and clinical application. Philadelphia, PA: Lippincott Williams and Wilkins. [Ref.]
- Krishnan A, Xu Y, Gandour J, Cariani P (2005) Encoding of pitch in the human brainstem is sensitive to language experience. Brain Res Cogn Brain Res 25: 161-168. [Ref.]
- Lobarinas E, Spankovich C, Le Prell CG (2017) Evidence of “hidden hearing loss” following noise exposures that produce robust TTS and ABR wave-I amplitude reductions. Hear Res 349: 155-163. [Ref.]
- Kumar UA, Ameenudin S, Sangamanatha AV (2012) Temporal and speech processing skills in normal hearing individuals exposed to occupational noise. Noise Health 14: 100-105.
- Ruggles D, Bharadwaj H, Shinn-Cunningham BG (2012). Why middle-aged listeners have trouble hearing in everyday settings. Curr Biol 22: 1417-1422. [Ref.]
- Epp B, Hots J, Verhey JL, Schaette R (2012) Increased intensity discrimination thresholds in tinnitus subjects with a normal audiogram. J Acoust Soc Am 132: 196-201. [Ref.]
- Bernstein LR, Trahiotis C (2016) Behavioral manifestations of audiometrically-defined “slight” or “hidden” hearing loss revealed by measures of binaural detection. J Acoust Soc Am 140: 3540. [Ref.]
- Bharadwaj HM, Verhulst S, Shaheen L, Liberman MC, ShinnCunningham BG (2014) Cochlear neuropathy and the coding of supra-threshold sound. Front Syst Neurosci 8: 26. [Ref.]
- Sanchez TG, Moraes F, Casseb J, Cota J, Freire K, et al. (2016) Tinnitus is associated with reduced sound level tolerance in adolescents with normal audiograms and otoacoustic emissions. Scientific Reports 6: 27109. [Ref.]
- King A, Hopkins K, Plack CJ (2013) Differences in short-term training for interaural phase difference discrimination between two different forced-choice paradigms. J Acoust Soci Am 134: 2635-2638. [Ref.]
- Pienkowski M (2017) On the Etiology of Listening Difficulties in Noise Despite Clinically Normal Audiograms. Ear Hear 38: 135-148. [Ref.]
- Fried MP, Dudek SE, Bohne BA (1976) Basal turn cochlear lesions following exposure to low-frequency noise. Trans Sect Otolaryngol Am Acad Ophthalmol Otolaryngol 82: 285-298. [Ref.]
- Badri R, Siegel JH, Wright BA (2011) Auditory filter shapes and high-frequency hearing in adults who have impaired speech in noise performance despite clinically normal audiograms. J Acoust Soc Am 129: 852-863. [Ref.]
- Schaefer SD, Post JD, Close LG, Wright CG (1985) Ototoxicity of low- and moderate-dose cisplatin. Cancer 56: 1934-1939. [Ref.]
- Fausti SA, Larson VD, Noffsinger D, Wilson RH, Phillips DS, et al. (1994) High-frequency audiometric monitoring strategies for early detection of ototoxicity. Ear Hear 15: 232-239. [Ref.]
- Knight KR, Kraemer, DF, Winter C, Neuwelt EA (2007) Early changes in auditory function as a result of platinum chemotherapy: Use of extended high-frequency audiometry and evoked distortion product otoacoustic emissions. J Clin Oncol 25: 1190-1195. [Ref.]
- American Speech-Language-Hearing Association (2018) Audiologic Management of Individuals Receiving Cochleotoxic Drug Therapy. [Ref.]
- Kwang Kyu Yu, Chi Ho Choi, Yong-Hwi An, Min Young Kwak, Soo Jung Gong, et al. (2014) Comparison of the Effectiveness of Monitoring Cisplatin-Induced Ototoxicity with Extended High-Frequency Pure-Tone Audiometry or Distortion-Product Otoacoustic Emission. Korean J Audiol 18: 58-68. [Ref.]
- Gorga MP, Neely ST, Ohlrich B, Hoover B, Redner J, et al. (1997) From laboratory to clinic: a large scale study of distortion product otoacoustic emissions in ears with normal hearing and ears with hearing loss. Ear Hear 18: 440-455. [Ref.]
- Hoben R, Easow G, Pevzner S, Parker MA (2017) Outer Hair Cell and Auditory Nerve Function in Speech Recognition in Quiet and in Background Noise. Frontiers in Neuroscience 11: 157. [Ref.]
- Valero MD, Hancock KE, Liberman MC (2016) The middle ear muscle reflex in the diagnosis of cochlear neuropathy. Hear Res 332: 29-38. [Ref.]
- American Speech-Language-Hearing Association (2005) (Central) Auditory Processing Disorders. Technical Report. [Ref.]
- Musiek FE, Chermak GD (2014) Handbook of Central Auditory Processing Disorder. In: Auditory Neuroscience and Diagnosis. 3rd edition, San Diego, Plural Publishing. [Ref.]
- Lopez-Poveda EA, Barrios P (2013) Perception of stochastically undersampled sound waveforms: A model of auditory deafferentation. Front Neurosci 7: 124. [Ref.]
- Amitay S, Ahissar M, Nelken I (2002) Auditory processing deficits in reading disabled adults. J Assoc Res Otolaryngol 3: 302-320. [Ref.]
- Loo JH, Bamiou DE, Rosen S (2013) The impacts of language background and language-related disorders in auditory processing assessment J Speech Lang Hear Res 56: 1-12. [Ref.]
- Skoe E, Kraus N (2010) Auditory brain stem response to complex sounds: a tutorial. Ear Hear 31: 302-324. [Ref.]
- Song JH, Skoe E, Banai K, Kraus N (2011) Perception of speech in noise: neural correlates. J Cogn Neurosci 23: 2268-2279. [Ref.]
- Riccio CA, Reynolds CR, Lowe PA (2001) Clinical Applications of Continuous Performance Tests: Measuring Attention and Impulsive Responding in Children and Adults. Hoboken, Wiley. [Ref.]
- Starr A, Picton TW, Sininger Y, Hood LJ, Berlin CI, et al. (1996) Auditory neuropathy. Brain 119: 741-754. [Ref.]
- Ferraro JA, Krishnan G (1997) Cochlear potentials in clinical audiology. Audiol Neurootol 2: 241-256. [Ref.]
- Nadol JB Jr, Thornton AR (1987) Ultrastructural findings in a case of Meniere’s disease. Ann Otol Rhinol Laryngol 96: 449-54. [Ref.]
- Stankovic K, Rio C, Xia A, Sugawara M, Adams JC, Liberman MC, et al. (2004) Survival of adult spiral ganglion neurons requires erbB receptor signaling in the inner ear. J Neurosci 24: 8651-8661. [Ref.]
- Wan G, Gomez-Casati ME, Gigliello AR, Liberman MC, Corfas G, et al. (2014) Neurotrophin-3 regulates ribbon synapse density in the cochlea and induces synapse regeneration after acoustic trauma. eLife 3: e03564. [Ref.]
- Sly DJ, Campbell L, Uschakov A, Saief ST, Lam M, et al. (2016) Applying neurotrophins to the round window rescues auditory function and reduces inner hair cell synaptopathy after noiseinduced hearing loss. Otol Neurotol 37: 1223-1230. [Ref.]
- zuki J, Corfas G, Liberman MC (2016) Round-window delivery of neurotrophin 3 regenerates cochlear synapses after acoustic overexposure. Sci Rep 6: 24907. [Ref.]
- Hill K, Yuan H, Wang X, Sha SH (2016) Noise-Induced Loss of Hair Cells and Cochlear Synaptopathy Are Mediated by the Activation of AMPK. J Neurosci 36: 7497-7510. [Ref.]
- de Villers-Sidani E, Alzghoul L, Zhou X, Simpson KL, Lin RC, et al. (2010) Recovery of functional and structural age-related changes in the rat primary auditory cortex with operant training. Proc Natl Acad Sci U S A 107: 13900-13905. [Ref.]
- Whitton JP, Hancock KE, Polley DB (2014) Immersive audiomotor game play enhances neural and perceptual salience of weak signals in noise. Proc Natl Acad Sci 111: E2606-E2615. [Ref.]
- Zheng W (2012) Auditory map reorganization and pitch discrimination in adult rats chronically exposed to low-level ambient noise. Front Syst Neurosci 6: 65. [Ref.]
- Moreno S, Bidelman GM (2014) Examining neural plasticity and cognitive benefit through the unique lens of musical training. Hear Res 308: 84-97. [Ref.]
- Noreña AJ, Chery-Croze S (2007) Enriched acoustic environment rescales auditory sensitivity. Neuroreport 18: 1251-1255. [Ref.]
- Schaette R, König O, Hornig D, Gross M, Kempter R, et al. (2010) Acoustic stimulation treatments against tinnitus could be most effective when tinnitus pitch is within the stimulated frequency range. Hear Res 269: 95-101. [Ref.]
- Cameron S, Glyde H, Dillon H (2012) Efficacy of the LiSN & Learn auditory training software: Randomized blinded controlled study. Audiol Res 2: e15. [Ref.]
- Roup CM, Post E, Lewis J (2017) Mild-Gain Hearing Aids as a Treatment for Adults with Self-Reported Hearing Difficulties. J Am Acad Audiol.
- Surr RK, Montgomery AA, Mueller HG (1985) Effect of amplification on tinnitus among new hearing aid users. Ear Hear 6: 71-75. [Ref.]
- Del Bo L, Ambrosetti U (2008) Hearing aids for the treatment of tinnitus. Prog Brain Res 166: 341-345. [Ref.]
- Ward ED, Cushing EM, Burns EM (1976) Effective quiet and moderate TTS: Implications for noise exposure standards. Acoustical Society of America 59: 160-165. [Ref.]
- Henderson D, Hamernik RP (1986) Impulse noise: critical review. J Acoust Soc Am 80: 569-584. [Ref.]
Download Provisional PDF Here
Article Type: REVIEW ARTICLE
Citation: Zheng Y, Guan J (2018) Cochlear Synaptopathy: A Review of Hidden Hearing Loss. J Otorhinolaryngol Disord Treat 1(1): dx.doi.org/10.16966/jodt.105
Copyright: © 2018 Zheng Y, et al. This is an open-access article distributed under the terms of the Creative Commons Attribution License, which permits unrestricted use, distribution, and reproduction in any medium, provided the original author and source are credited.
Publication history:
SCI FORSCHEN JOURNALS
All Sci Forschen Journals are Open Access