Figure 1: Direct and indirect mechanisms in the pathogenesis of neuroCOVID symptoms.
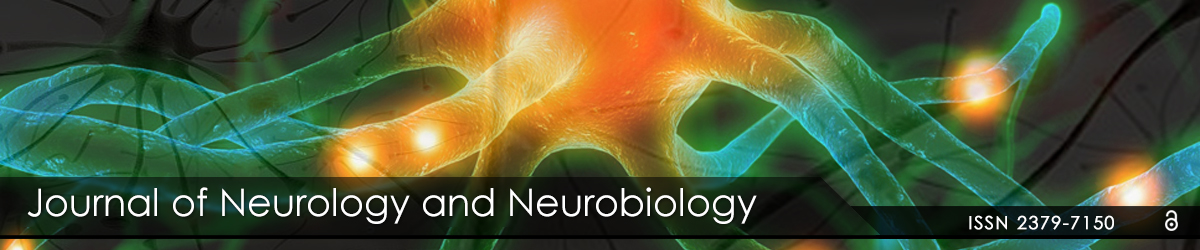
Full Text
Anna Rizzi1 Stefano Zoccolella2 Giovanni Iliceto1 Vincenzo Centonze3 Matteo Saccia1 Vincenzo Benagiano1*
1Department of Basic Medical Sciences Neuroscience and Sense Organs, University of Bari, Bari, Italy2Neurology Unit, ASL Bari, San Paolo Hospital, Bari, Italy
3Department of Biomedical Sciences and Human Oncology, University of Bari, Bari, Italy
*Corresponding author: Vincenzo Benagiano, Department of Basic Medical Sciences Neuroscience and Sense Organs, University of Bari, Bari, Italy, E-mail: vincenzo.benagiano@uniba.it
NeuroCOVID is a neuropsychiatric syndrome that overall occurs in 36%-84% of patients suffering from COVID-19 (corona virus disease-2019). The neuroCOVID symptoms are manifold and heterogeneous, show different levels of severity, and occur both in acute and subacute/chronic phase of the disease. Two types of neuropsychiatric symptoms can be distinguished on the basis of their pathogenesis: (i) symptoms due to the direct neuropathogenic effect of SARS CoV-2 (direct symptoms); (ii) symptoms related with indirect neuropathogenic effect of the virus, mediated by hypoxic/ischemic or by immunopathological mechanisms activated during SARS CoV-2 infection (indirect symptoms). Aim of this review was to analyze the main neuropsychiatric symptoms of the neuroCOVID and accurately examine, for each of them, facts and advanced hypotheses to explain their pathogenesis.
Severe acute respiratory syndrome coronavirus-2 (SARS CoV-2); Corona virus disease-2019 (COVID-19); Neurogenous and hematogenous route; Cytokine release syndromes; Autoimmune syndromes; LongCOVID
COVID-19 (corona virus disease-2019) is an acute infectious disease caused by the severe acute respiratory syndrome coronavirus-2 (SARS CoV-2), which has appeared in China in late 2019 and has rapidly spread worldwide provoking the outbreak of a pandemic. In most cases, COVID-19 is asymptomatic or mildly symptomatic, clinically manifesting as an influenza-like syndrome, but in 15-30% of cases, it is associated with an atypical pneumonia responsible for Severe Acute Respiratory Distress (ARDS) and with systemic complications [1-2]. The considerable variability of clinical manifestations of COVID-19, in terms of heterogeneity of symptoms, severity and relative frequency of each of them, must be related, at least partly, with the appearance during the pandemic of a number of variants of SARS CoV-2, which have led to significant changes in virus transmissibility, pathogenic power, virulence and ability to resist host defenses [3-5].
The term neuroCOVID has been introduced to indicate the set of neuropsychiatric symptoms present in some COVID-19 patients [6,7]. According to various reports of cases, the neuroCOVID would affect 36% to 84% of COVID-19 symptomatic patients [6- 9]. This excessive variability of frequency of neuropsychiatric symptoms in COVID-19 patients can only partially be correlated with real variability in the clinical manifestations of the disease. In fact, it is likely that the different countries have failed to adopt homogeneous and appropriate diagnostic measures that, ultimately, have led to not uniform collections of epidemiological data [10]. Moreover, a number of factors have contributed to make it difficult the collection of neuroCOVID symptoms: (i) the emergency health condition caused by the COVID-19 pandemic, especially at its early stage, has prevented from carrying out a clinical evaluation of all COVID-19 patients and, consequently, has not made possible an exhaustive collection of the neuroCOVID symptoms [11,12]; (ii) some common symptoms of COVID-19 (e.g., influenza-like and visceral ones) have been differently interpreted, being considered as symptoms of neuroCOVID, in some cases, and as symptoms of viral mucositis of unspecified origin affecting respiratory or alimentary tracts, in other cases [13]; (iii) some neuroCOVID symptoms have frequently been confused with those caused by neurological and psychiatric comorbidities, given the absence of a precise analysis of the patient’s clinical history and confirmation by appropriate diagnostic tests [14,15]; (iv) the scarce number of autopsies carried out on neuroCOVID patients has prevented obtaining data on the real frequency of neuropathological lesions in patients died from COVID-19 [16]. On the other hand, the variability of the clinical picture of neuroCOVID could depend with virus related factors and/or with host-related factors. Difference in neurotropism and neuropathogenicity of SARS CoV-2 could be related with the virus variant, although up to now it has not been ascertained whether exist virus variants that show greater or lesser capacity to damage the nervous system [17,18] (actually, in many cases, the variant present in a neuroCOVID patient is not even known). The host-related factors include congenital or acquired factors which increase the vulnerability of the nervous system to the actions of SARS CoV-2 [19-22]. Also the results of epidemiological studies reporting data on the frequency of neuroCOVID symptoms in hospitalized patients have shown some discrepancy: a study that collected separately symptoms referred by patients and signs assessed by clinical evaluation, has reported that the most common among the former are headache (37%) and anosmia/ ageusia (26%), and among the latter are acute encephalopathy (49%), coma (17%), stroke (6%) and diffuse encephalitis (0.5%) [9]; another study, in which no separation was made, has reported that the most common symptoms are headache (13.4%), acute encephalopathy (10.1%) and coma (9.7%) [23]. On the other hand, both these studies, conducted on hospitalized patients, have not provided information on the frequency of all neuroCOVID symptoms, since they have not taken into account some neuropsychiatric symptoms, such as anxiety or depression and dysautonomic symptoms, which are very common in neuroCOVID patients, but usually do not require hospitalization. At present, the available data on the epidemiology of neuroCOVID symptoms are incomplete, although the results of some multicenter retrospective-prospective observational studies have begun to be published [24,25].
On the basis of the pathogenesis, the neuroCOVID symptoms can be distinguished: in (i) symptoms deriving from direct pathogenic mechanisms, or direct symptoms; and (ii) symptoms deriving from indirect pathogenic mechanisms, or indirect symptoms. The former are clinical manifestation of pathogenic effects that SARS CoV-2 exerts on the human nervous tissues, thus attributable to virus neurotropism; the latter are clinical manifestations of nervous tissue damage caused by mechanisms that are independent of presence of SARS CoV-2 in the nervous tissues, possibly triggered by hypoxic/ischemic or immunopathological mechanisms arising in the course of SARS CoV-2 infection [26]. The neuroCOVID symptoms are manifold and heterogeneous and show different degrees of severity, according to the site of Central Nervous System (CNS) or of Peripheral Nervous System (PNS) where the lesions are localized, and the number and extent of the lesions [8,27-29].
Aim of this review was to supply an overview of the state of the art and advances on pathogenic mechanisms underlying the neuroCOVID symptoms. Starting from an in-depth analysis of data in literature on the clinical manifestations of neuroCOVID, on the findings obtained by COVID-19 patients subjected to imaging and neuropathological examinations, and on the results of in vitro and in vivo experimental studies, the pathogenic mechanisms for all the neuroCOVID symptoms have been critically reviewed. The pathogenic mechanisms have been described separately, distinguishing those from direct mechanisms from those from indirect ones, and identifying the different routes through which each mechanism takes places.
Undoubtedly, the data reported in this review have to be considered as preliminary, firstly because it is not always possible to know exactly the pathogenetic mechanism of a neuropsychiatric symptom: this could be due to the fact that the data available in the case reports often are not sufficient to clarify the pathogenesis, or that the mechanism is really difficult to identify since the pathogenesis can be due to multiple, variously associated causes.
The authors assume that the present review, in addition to supply updated information on the neurobiology of SARS CoV-2 and on its capacity to damage nervous tissues, could also provide a useful tool for medical practice for early and precise recognition of each neuropsychiatric symptom and for implementation of appropriate therapeutic strategies.
SARS COV-2 infection is principally contracted by airborne transmission and the virus primary localization is mainly represented by the mucosal membrane of the respiratory tracts and the pulmonary alveoli [30-32]. Less frequently, the primary localization is represented by mucosal membrane of the alimentary canal [33,34] and of the conjunctival sac [35], or by the integument [36]. The molecular basis for SARS CoV-2 attack to human cells is given by the docking of the spike-protein, present on viral surface, to the Angiotensinconverting Enzyme-2 (ACE-2), attached to membrane of the target cells. The subsequent entry into cell prevents the normal functioning of ACE-2, causing disruption of the Renin-angiotensin-aldosterone System (RAAS) homeostasis, and leads the cell to lysis or damage [37-39]. ACE-2 receptors are expressed by a wide range of human cells, including mature epithelial, connective, muscle and nerve cells [40,41] as well as stem cells [41,42]. In particular, they have been detected in ciliated epithelial cells of the mucosa of respiratory tracts and in epithelial alveolar cells (mainly type II pneumocytes), which usually are the first cells to be attacked by SARS CoV-2 [43,44]. To a lesser extent, ACE-2 receptors have been detected in epithelial cells of oro-pharyngo-esophageal and gastrointestinal mucosa [45,46], of conjunctival mucosa [47] and of integument [48], which are also possible target cells of the virus. After the primary localization, SARS CoV-2 can affect distant sites, so that COVID-19 assumes connotations of multisystem disease, associated with a polymorphic clinical picture affecting not only the respiratory system, but also the nervous, cardiovascular, urinary and endocrine system [1,2,6,8,9].
Among the distant sites, the nervous system is certainly one of most frequently affected through direct and indirect mechanisms.
Direct mechanisms
The direct mechanisms are supported by interaction between SARS CoV-2 and ACE-2 receptors expressed by a number of neurons and gliocytes [49-54]. These ACE-2 expressing neurons have been seen extensively distributed in brain, including telencephalon (cerebral cortex), diencephalon (hypothalamus) and brainstem (medulla oblongata tegmentum) [55,56], particularly in centers involved in the nervous regulation of the cardiovascular, respiratory and endocrine system [56]. The molecular basis for SARS CoV-2 attack has been sufficiently proven by in vitro and in vivo experiments [52,57]. It would be exerted through the following steps: (i) invasion of nerve fiber endings (neurogenous route) or cellular constituents of capillaries (hematogenous route), in the site of primary localization; (ii) reaching CNS running along peripheral nerves (neurogenous route) or by the bloodstream (hematogenous route); (iii) invasion of CNS tissues by transneuronal diffusion (neurogenous route) or by crossing CNS blood capillary wall (hematogenous route); (iv) attack of virus-sensitive nervous cells. Actually, none of the above steps has been ascertained in human. In any case, the detection in the human brain of lesions with positivity for SARS CoV-2 RNA, using Reverse Transcriptase Polymerase Chain Reaction (RT-PCR), and for SARS CoV-2 expressing antigens (e.g., spike protein, nucleocapsid protein), using immunohistochemistry, are elements that suggest the existence of the direct neuropathogenic mechanisms [58].
Neurogenous route (Figure 1): Experimental studies have reported that coronaviruses introduced in the nasal mucosa are detectable, shortly after the introduction, in both PNS and CNS tissues [59,60]. Actually, the presence of ACE-2 receptors on nerve endings in the mucosal membranes, which is the prerequisite for the neurogenous route, has been only occasionally reported [61]. In general, the precise mechanisms underlying the transmucosal diffusion, involving the virus transport along nerve fibers, the invasion of the CNS tissues, involving the virus transneuronal transfer and its progressive spread in ever larger zones of the brain, involving local diffusion or distant propagation via central tracts or Cerebrospinal Fluid (CSF), are really poorly known in human [62-64]. In addition, the detection tests for SARS CoV-2 markers on postmortem brain samples collected from neuroCOVID patients have frequently given negative results, suggesting that the direct mechanisms are not constantly used by the virus to invade the nervous tissues [58,65,66].
Actually, two main neurogenous routes have been claimed: the olfactory route and the vagal route. Additional neurogenous routes have also been postulated, but would be a rare occurrence.
Olfactory route: ACE-2 receptors have been abundantly detected in epithelial cells and, to a lesser amount, in olfactory neurons of the olfactory mucosa [67-69]. After attack of epithelial cells or, directly, of olfactory neurons, SARS CoV-2 would pass in olfactory nerve fibers and transported retrogradely up to the olfactory bulb; alternatively, the virus would progress through vessels accompanying the olfactory nerves or through the perineural spaces surrounding them [62]. Experimental studies on SARS CoV-2 infected mouse have indicated that the olfactory route is probably the main route used by the virus to enter the CNS and the olfactory bulb is the brain region where the virus is localized most frequently [59]. The further spreading of the virus would happen along the olfactory tract and the medial and lateral olfactory striae, through which it can reach the olfactory areas of the cerebral cortex and the septal nuclei [70].
Neuropathological studies carried out on postmortem samples from neuroCOVID patients have confirmed focal encephalitis localized in the olfactory regions, histologically characterized by intense astrogliosis and microgliosis infiltrates of lymphocytes and marked neuronal degeneration, in most cases positive to detection tests for SARS CoV-2 markers [58,71]. Electron microscopy observations carried out on postmortem samples of the olfactory bulb have provided ultrastructural evidence for presence of SARS CoV-2 particles within neurons or scattered in the neuropil [72].
Vagal route: In this route, the cell target of SARS CoV-2 are the ACE-2 expressing epithelial cells in the mucosal membranes of upper and lower respiratory tract, in the pulmonary alveoli and, to a lesser extent, in the mucosal membranes of alimentary canal [44-47]. It would follow the transfer of the virus from the epithelial cells to the free nerve endings and its transport along vagal nerve fibers to its nuclei in the medulla oblongata tegmentum, namely the nucleus of solitary tract, the dorsal motor nucleus of vagal nerve and the nucleus of spinal tract of trigeminal nerve; at a later stage, the virus could spread from these nuclei to the surrounding reticular formation, eventually affecting the full brainstem tegmentum, the cerebellum and the diencephalon [73,74]. The existence of the vagal route appears in agreement with the demonstration of high number of ACE-2 expressing neurons in the medullary tegmentum [51,53], and the frequent finding of focal encephalitis with positivity for SARS CoV-2 markers in postmortem samples of medullary tegmentum from neuroCOVID patients [58,71].
Other neurogenous routes: Additional neurogenous routes have been suggested. The gustatory route would be based on the docking of SARS CoV-2 to ACE-2 receptors of the gustatory mucosa [45]; subsequent virus invasion of the nerve endings in the gustatory mucosa and its progression to the brain along fibers of the intermedius, glossopharyngeal and vagal nerve would probably progress similarly to the vagal route. The trigeminal route would follow a primary localization of SARS CoV-2 in conjunctival, nasal (non-olfactory) and oral (non-gustatory) mucosa [33-35] and reach the brain along nerve fibers of the trigeminal nerve: demonstration of ACE-2 receptors in the trigeminal ganglion [75] and SARS CoV-2 markers in the trigeminal nuclei [66] would support the existence of this route. Finally, the spinal nerve route has been suggested to explain the central diffusion of SARS CoV-2 in cases of primary localization in the gastrointestinal mucosa [33], through the splanchnic nerves, and in the integument [36], through cutaneous branches of the spinal nerves; this suggestion could be supported by the detection of ACE-2 receptors in the dorsal root ganglia of spinal nerves [76] and by the finding of cases of spinal myelitis (acute transverse myelitis) positive for SARS CoV-2 markers [77,78].
Hematogenous route (Figure 1): The simple observation that brain lesions caused by SARS CoV-2 can localize in any region of it implies the existence of the hematogenous route. This route would be activated by the virus docking to ACE-2 receptors expressed by the cellular components of the wall of capillaries in the site of primary localization and the subsequent crossing of the capillary wall and invasion of the bloodstream (viremia). The invasion of the nervous tissues would be determined by the virus docking to ACE-2 receptors on the components of CNS capillaries and their crossing. Although the hematogenous route is widely accepted [79,80], data demonstrating the existence of this route are largely incomplete in humans. For example, the data on the expression of ACE-2 receptors in the blood vessel wall are controversial: although they have been observed in endothelial cells of arteries and veins [39,40], their demonstration on endothelial cells of CNS capillaries, indispensable element to support the hematogenous route, has been only occasionally provided [81]. Evidence exists that SARS CoV-2 is not able or have minimal capacity to cross the Blood Brain Barrier (BBB), and, therefore, the diffusion through capillary wall take places only in brain regions where the BBB is physiologically absent, such as the Circumventricular Organs (CVO), i.e. subfornical organ, vascular organ of the lamina terminalis, median eminence and area postrema [82-84]. Experimental studies have evidenced the SARS CoV-2 spike protein exerts deleterious effects on primary cultures of human brain microvascular endothelial cells resulting in loss of BBB functional integrity [85]: this datum could supply the pathophysiological basis for the hematogenous invasion of CNS regions with less selective BBB, such as the medulla oblongata tegmentum. All the brain regions with absent or less selective BBB are those where inflammatory lesions with positivity for SARS CoV-2 markers have been more frequently found [58,71,86,87].
On the other hand, brain regions affected by chronic inflammatory or degenerative diseases that cause loss of functional integrity of BBB seem to be more susceptible to be invaded by SARS CoV-2 through the hematogenous route. This is suggested by clinical studies reporting an increased frequency of diffuse encephalitis with positivity for SARS CoV-2 in patients with Multiple Sclerosis (MS) or with Alzheimer’s Disease (AD) [88,89]. Finally, it has been suggested that SARS CoV2 may invade the CNS tissues traveling inside circulating immune cells attacked by the virus; this ‘Trojan horse’ mechanism would be facilitated by the fact that the infected cells are better able to cross the BBB [49,60]. Finally, also the hypertension may cause BBB impairment and therefore enhance the risk of CNS invasion through the hematogenous route [90]. All these data indicate contributing causes that reduce BBB selectivity are necessary for the virus to invade the CNS through the hematogenous route. This would explain why inflammatory lesions with positive SARS CoV-2 are rarely found in brain regions where the BBB is intact.
Neuropathological studies have indicated that there is a correlation between the blood vascularization and the frequency of SARS CoV-2-positive encephalitis. The most vascularized regions (e.g., cerebral cortex, basal nuclei, internal capsule, diencephalon and cerebellum) are among the most frequently affected in the course COVID-19 [18].
The indirect mechanisms are independent of the localization of SARS CoV-2 in the nervous tissues. The damaging effects on the CNS are secondary to those exerted by SARS CoV-2 on the respiratory and the cardiovascular system, hypoxic/ischemic mechanisms, or on the immune system, immunopathological mechanisms. The CNS lesions caused by indirect mechanisms vary depending on the pathogenic mechanisms, but usually consist in diffuse encephalitis with negativity for SARS CoV-2 markers [91].
Hypoxic/ischemic mechanisms (Figure 1)
Hypoxic/ischemic mechanisms are related with the hypoxemia caused by SARS CoV-2 pneumonia or with the impaired CNS blood flow caused by vasculitis, cardiopathy, or hemocoagulation disorders associated with SARS CoV-2 infection [92-96]. Due to hypoxia, nervous tissues undergo widespread suffering that results in the Diffuse Hypoxic Encephalitis (DHE), neuropathologically characterized by massive vasodilation, edema and neuronal lost [85,87,97]. Since the lesions are produced through indirect mechanisms, postmortem samples from COVID-19 patients with DHE usually give negative results to detection tests for SARS CoV-2 markers [58]. The earliest and most heavily affected CNS regions are those with most intense neuronal activity and higher metabolic oxygen and glucose demands, including the telencephalon, the diencephalon and the cerebellum [86,87,97].
In addition, single or multiple infarctions, especially localized in the internal capsule or in the centrum semiovale, have been observed in association with diffuse encephalitis or as only neuropathological finding [58,71,98,99]. Actually, it is unclear whether the infarctions are directly related with the viral infection. COVID-19 patients suffering from cerebral infarction generally present cardiovascular risk factors, including advanced age, hypertension, hypercoagulability, diabetes, and medical history of cerebrovascular disease, which are all factors that predispose to the onset of infarction and are independent of the current SARS CoV-2 infection [100-102]. On the other hand, epidemiological studies have indicated that the frequency of cerebral infarction is increased in COVID-19 patients [9].
Another possible picture possibly linked to hypoxic/ischemic mechanisms, detected in some COVID-19 patients undergoing MRI examinations may consist of diffuse edema localized in the white matter of both the parietal lobe, responsible for the so-called Posterior Reversible Encephalopathy Syndrome (PRES) [103,104].
Immunopathological mechanisms (Figure 1)
The immunopathological mechanisms are basically represented by cytokine-release syndromes and autoimmune syndromes.
Cytokine-release syndromes
ACE-2 expressing hematopoietic stem cells in the bone marrow is a possible target of SARS CoV-2 [42]. It has been stated that the infected stem cells may give rise to progenies of lymphocytes and macrophages responsible for dysregulated synthesis and release of chemical mediators of immune response and inflammation [105,106]. Accordingly, increased serum levels of inflammatory cytokines have been detected in COVID-19 patients, including Interleukin-2 (IL-2), Interleukin-6 (IL-6), Interleukin-7 (IL-7), Granulocyte-macrophage Colony-stimulating Factor (GM-CSF), Interferon γ (IFN γ), IFN γ-induced Protein-10 (IP-10), Monocyte Chemoattractant Protein-1 (MCP-1), Macrophage Inflammatory Protein-1α (MIP-1α) and tumor necrosis factor- α (TNF-α) [107]. Among the substances increased in COVID-19, is the MCP-1, a chemokine responsible for the transmigration of the monocytes across the BBB [108]: this finding has supported the hypothesis that a possible route for CNS invasion by SARS CoV-2 is mediated by circulating monocytes [50].
The release of cytokines is a defense mechanism that intervenes in the course of SARS CoV-2 infection and is responsible for the onset of influenza-like symptoms, including headache, arthralgias, myalgias, asthenia, fatigue and general malaise, which are frequently present in the course of COVID-19 [1,2]. In some cases, the release of cytokines is strikingly intense, assuming features of the so-called cytokine storm, which represents a severe, fatal complication of COVID-19. It is a systemic inflammatory response syndrome, characterized by inflammatory processes possibly localized in all organs, including the encephalon [109].
The neuropathological findings related with the cytokinerelease syndrome are variable, ranging from a modest infiltrates of lymphocytes and monocytes with minimal or absent phenomena of neuronal suffering to massive inflammatory infiltrates, intense reactive astrogliosis and microgliosis (formation of microglial nodules), hemorrhagic foci and massive neuronal loss [58,18]. In the latter case, it is referred to as Diffuse Cytokine-Release Encephalitis (DCRE). Immunohistochemical staining for the astrocytic marker, Glial Fibrillary Acid Protein (GFAP), has confirmed variable degrees of astrogliosis; that for the microglia marker, Human Leukocyte Antigen DR isotype (HLA-DR), has shown high degree of microgliosis; that for the cytotoxic T-lymphocyte marker CD-8 has revealed increase of CD8-positive lymphocytes [110]. However, the most relevant datum is the almost constant negativity to the detention tests for SARS CoV2 markers in postmortem samples of nervous tissues and in CSF [58,71,18]. Since DCRE causes significant reduction of BBB selectivity, it facilitates the virus invasion of the brain, possibly of every region of it [111], causing an encephalitis by direct mechanisms with positivity for SARS CoV-2, which, in extreme cases, it may lead to a diffuse encephalitis.
In addition, the cytokine-release syndrome present in COVID-19 has been associated with hemocoagulation disorders responsible for thromboembolic phenomena and Disseminated Ontravascular Coagulation (DIC) [112,113]. The genesis of these phenomena is probably multifactorial, as it may depend on concomitant vasculitis and cardiopathy [92-96]. However generated, they can lead to the formation of cerebral infarction or hemorrhage or the onset of diffuse ischemic encephalopathy [58].
Finally An acute multi-systemic vasculitis with possible involvement of cerebral arteries has been described in pediatric and adolescent COVID-19 patients, displaying clinical and anatomopathological features similar to those of the Mucocutaneous Lymph Node Syndrome (MLNS), or syndrome of Kawasaki; since this syndrome is caused by uncontrolled immune response to common antigenic stimuli in genetically predisposed subjects, it has been suggested that similar mechanisms could intervene during COVID-19 [114,115].
Autoimmune syndromes
Dysregulation of immune mechanisms related with SARS CoV-2 infection may also consist of the triggering of autoimmune response. This would be supported by mechanisms of molecular mimicry, based on molecular similarity between virus antigens and nervous tissue antigens, or by mechanisms of bystander activation, based on nonspecific activation of T-lymphocytes that facilitates the activity of natural killer cells, the release of cytolytic molecules and, finally, the production of autoantibodies [116]. Autoimmune mechanisms have been suggested for the pathogenesis of nervous lesions affecting the CNS and the PNS.
As regards the CNS lesions, it has been hypothesized that SARS CoV-2 may act as priming infectious agent of central demyelination processes, similar to those present in MS, on the basis of the detection of autoreactive T lymphocytes able to recognize both human coronaviruses and myelin antigens [117] and of autoantibodies in the CSF of COVID-19 patients [118]. On the other hand, symptoms of neuroCOVID and of MS can associate in case of COVID-19 and MS comorbidity, and significantly interfere with each other, in general causing a reciprocal aggravation [119].
As regards the PNS lesions, autoimmune mechanisms have been hypothesized for the pathogenesis of acute peripheral neuropathies attributable to production autoantibodies against myelin and/or Schwann cell antigens. This hypothesis has been supported by a number of observations carried out on COVID-19 patients suffering from Guillain-Barré-Strohl Syndrome (GBSS): (i) SARS CoV-2 has never been detected either in the peripheral nerves or in the CSF [120]; (ii) anti-spike protein antibodies showing molecular mimicry for nerve gangliosides or other nerve antigens have been detected [121-123]; (iii) patients have responded positively to Intravenous Immunoglobulin (IVIg) therapies [121]. Finally, the pathogenic link between COVID-19 and GBSS has been supported by the observation of significant increase of incidence of GBSS during the COVID-19 pandemic [124]. However, the actual correlation between COVID-19 and GBSS is a controversial area and some scholars have expressly denied this correlation [125,126].
Neuropathological lesions related with SARS CoC-2 are topographically distinguished in central and peripheral (Table 1).
Localization | Neuropathological Finding | Response to SARS CoV-2 Tests | Neuropathogenic Mechanisms | Main Symptoms |
CNS | ||||
Telencephalon:
|
Focal Encephalitis | Positivity |
Direct Mechanism:
|
Olfaction Disorders |
Brainstem: Medullary Tegmentum
|
Focal Encephalitis | Positivity |
Direct Mechanism:
|
Dysautonomic Disorders:
|
Diencephalon: Hypothalamus | Focal Encephalitis | Positivity |
Direct Mechanism:
|
Dysautonomic Disorders:
|
Telencephalon:
|
Diffuse Encephalitis Focal Encephalitis Infarction Hemorrage | Negativity Positivity Negativity | Indirect Mechanism:
|
Influenza-like Symptoms Psychic Symptoms:
|
Cerebellum:
|
Focal Encephalitis | Positivity |
Direct Mechanism:
|
Cerebellar symptoms |
PNS | ||||
GBSS | Polyradiculoneuritis of Spinal Nerves | Negativity | Indirect Mechanism:
|
Spinal Nerve Symptoms |
MFS | Polyradiculoneuritis of Cranial Nerves | Negativity | Indirect Mechanism:
|
Cranial Nerve Symptoms |
Table 1: Synoptic view of the most common neuropathoplgical lesions in patients with neuroCOVID: localization, pathological anatomy, pathogenic mechanism and most frequent clinical manifestations.
Central lesions
The CNS regions most often affected include (in order of frequency): the olfactory regions of the telencephalon, the medulla oblongata tegmentum, the hypothalamus, the remaining telencephalon, and the cerebellum.
Olfactory regions: The olfactory bulb is probably the main CNS target of SARS CoV-2, firstly reached through the olfactory route [58]. Further diffusion in the CNS would happen through the olfactory tract and the medial and lateral olfactory striae, through which the virus may affect the olfactory areas of cerebral cortex (entorhinal area, prepyriform area, uncus and limen insulae), the septal nuclei, the amygdala and the hypothalamus [70]. Computerized Tomography Imaging (CTI) or Magnetic Resonance Imaging (MRI) examinations carried out in neuroCOVID patients have frequently shown areas of hyperdensity or of hyperintensity in all these regions [99], and neuropathological investigations carried out on postmortem samples from neuroCOVID patients have confirmed frequent presence of focal encephalitis localized in the olfactory regions [58,71,18].
The contemporary inflammation of the olfactory regions as well as of the olfactory mucosa and of the olfactory nerve explains why the olfaction disorders are very common symptoms of neuroCOVID, so much to be considered as pathognomonic symptoms of COVID-19 [127,128]. Clinical studies based on functional-MR and Positron Emission Tomography (PET) techniques have suggested that quantitative olfaction deficit, hyposmia/anosmia (i.e., reduction/loss of the ability to detect smells), are prevalently symptoms of peripheral origin, while qualitative deficit, dysosmia (i.e., inability to recognize a smell or to confuse smells), are prevalently of central origin, therefore disturbances of olfactory perception [128-131].
Medulla oblongata tegmentum: Through the vagal route, and probably through the gustatory and trigeminal routes, SARS CoV-2 is able to invade the medulla oblongata tegmentum and progressively its neighboring regions [66,73,98]. The area postrema and, to a lesser extent, the nucleus of solitary tract itself, where the BBB is absent or less selective, may be invaded through hematogenous route [82-84]. MRI and neuropathological studies have frequently confirmed in neuroCOVID patients presence of lesions localized in all these regions [58,71,98].
Lesions in medullary tegmentum may affect autonomic centers involved in parasympathetic regulation of several visceral functions. Respiratory symptoms (cough, dyspnea) or digestive symptoms (vomiting, nausea, diarrhea), usually ascribed to inflammation of the mucosal membranes site of virus primary localization, may be due or made worse by lesions affecting medullary autonomic centers nervous [132,133]. Therefore, the above visceral symptoms should be considered, at least partly, as expression of central Therefore it should always be useful to keep in mind that such symptoms may be expression of central dysautonomic disorders, which can have serious consequences for the patient. In particular, the involvement of the respiratory centers, localized in the dorsal reticular formation of medulla (gigantocellular nucleus) and in the dorsolateral reticular formation of pons (parabrachial nuclear complex), may aggravate the patient’s respiratory failure, often already severely compromised by the SARS CoV-2 pneumonia [74,134]. The involvement of the vasomotor centers, localized in the ventrolateral reticular formation of caudal pons (parvocellular nucleus) may cause depression of heart activity and lowering of blood pressure, which can lead the patient to circulatory failure [135]. The involvement of the area postrema, site of the Chemoreceptor Trigger Zone (CTZ), localized in the medulla tegmentum near the floor of fourth ventricle, may cause the onset of incoercible vomiting associated with nausea and loss of appetite [136].
Other common, pivotal symptoms in COVID-19 patients are the taste disorders [19,129,137], comprising quantitative deficits, hypogeusia/ageusia (i.e., reduction/loss of the ability to detect tastes), and qualitative deficits, dysgeusia (perception of altered or unpleasant flavors). They may have peripheral or central origin. The disorders of peripheral origin would be due to the inflammation of the gustatory mucosa and nerves [129], whereas those of central origin would be due to virus invasion of the nucleus of solitary tract, through the gustatory route [45] or even of the superior nervous centers involved in the gustatory function (hypothalamus, gustatory area of the cerebral cortex, i.e., frontal operculum, temporal pole and anterior insula). Since the taste is conditioned by the olfaction, it has been is suggested that the taste disorders could actually be secondary to the olfaction ones [138].
Hypothalamus: Routes to invade the hypothalamus are the hematogenous route [139] and the propagation by continuity from the olfactory regions or the brainstem tegmentum [70,72,74]. Moreover, the hypothalamus may be affected by indirect hypoxic/ischemic and immunopathological mechanisms [140]. All hypothalamic nuclei are susceptible to be affected, with a preference for the nuclei of the median eminence (arcuate nucleus), due to the fact that the median eminence falls within the CVO [82-84] and that these nuclei contain neurons highly sensitive to inflammatory cytokines [80,141].
The onset of hypothalamic symptoms greatly contributes to enrich the clinical picture of the neuroCOVID. They include dysautonomic and limbic disorders: the former affect the respiratory function, the blood circulation, the homeostasis of body fluids, the metabolism and the hematolymphopoietic function; the latter include behavioral disorders affecting the eating or sexual behaviors, anxiety, depression and emotion disturbances, and sleep-wake cycle disorders [132,142- 145].
Telencephalon: With the exception of the already discussed olfactory regions, which are telencephalic regions mainly invaded through the neurogenous route, other regions of the telencephalon are mainly affected by indirect, hypoxic/ischemic or immunopathological mechanisms; the most affected regions are the cerebral cortex (neocortex), the hippocampus (archicortex), the white matter (corpus callosum, lamina terminalis, centrum semiovale, capsules) and the basal nuclei, often affected in association, in the context of a DHE or DCRE [58,86,87,18,97-100,146]. Other centers affected are the telencephalic CVO (subfornical organ, vascular organ of the lamina terminalis), which however are invaded through the hematogenous route [82-84].
The clinical symptomatology of diffuse encephalitis is extremely heterogeneous depending on location and extent of lesions. Symptoms include: cognitive impairment, disturbances of the attention, of the concentration and of the ideation, and memory loss; consciousness disorders of qualitative type (acute encephalopathy or delirium) and quantitative type (coma); sensory perception disorders affecting exteroception, proprioception, vestibular sensitivity, hearing and vision; motor disorders, such as seizures, muscle paralysis, spasticity, hypertonia and tremor, and defects of motor planning, including aphasia, apraxia, dysphagia; affective disturbances) [8,147].
In case of cerebral infarction or hemorrhage, symptoms arise as a stroke, characterized by hemiplegia, hemianesthesia, aphasia, acute encephalopathy and coma [147]. The spontaneous resolution of symptoms with full anatomical and functional recovery indicates that in some cases, the cause of stroke is due to Transient Ischemic Attack (TIA) or by Reversible Ischemic Neurologic Deficit (RIND) [148]. In case of PRES, the main symptoms are headache, seizures, acute encephalopathy and, characteristically, visual loss [103,104].
Finally, In case of isolated affection of the telencephalic CVO, disorders of body-fluid homeostasis have been reported [149].
Cerebellum: Lesions of the cerebellum occur through direct mechanisms (propagation by continuity, invasion by hematogenous route) or indirect mechanisms (DHE, DCRE). The cerebellar lesions (cerebellitis) have been found mainly in the medullary center, with possible involvement of the cerebellar cortex and nuclei [58,18,98,150,151].
Clinical examinations have frequently reported in COVID-19 patients cerebellar symptoms, including impairment of complex, coordinated and precise movements (asynergia, ataxia, apraxia, dysarthria, agraphia, dysmetria), disorders of automatic movements (gait and gaze disorders), disturbance of balance in static conditions and during walking [152]. Moreover, according to recent views indicating a role of cerebellum in the regulation of the cerebral cortex, through the cerebrocerebellar circuit, and of the hypothalamic nuclei, through the hypothalamocerebellar circuit [153,154], it has been hypothesized that the previously described sensorimotor, cognitive, affective and dysautonomic disorders, usually considered as of telencephalic or hypothalamic origin, may have a pathogenic component of cerebellar origin.
Peripheral lesions
As stated before, the neurogenous route implies firstly an invasion by SARS CoV-2 of peripheral nerves. In particular, the peripheral nerves most frequently affected are the olfactory and the vagal nerve. Infiltrates of lymphocytes and monocytes with reactive numerical increase of Schwann cells and signs of axon suffering have been observed in rare postmortem samples of olfactory nerve taken from neuroCOVID patients [155].
In the course of COVID-19, peripheral nerves may be affected through indirect mechanisms, mainly represented by autoimmune mechanisms [116]. These mechanisms have been invoked for the pathogenesis of a polyneuropathy affecting peripheral nerves (Table 1). In most cases, the polyneuropathy occurs clinically as a variant of the GBSS, generally as the acute inflammatory demyelinating polyradiculoneuropathy, but also as the acute motor axonal neuropathy and the acute motor sensory axonal neuropathy [121].
They comprise a spectrum symptoms characterized by acute ascending motor weakness, moderate sensory abnormalities and muscle or radicular pain in the spinal periphery [156,157]. More rarely, the polyneuropathy manifests clinically as the Miller-Fisher Syndrome (MFS), clinically characterized by facial nerve palsy, ophthalmoplegia, facial anesthesia/paresthesia, vestibular, hearing and visual disorders [158].
The post-COVID-19 condition (commonly indicated as longCOVID) is a clinical syndrome characterized by the persistence of clinical symptoms of COVID-19 for 4-12 weeks (subacute longCOVID) or for more than 12 weeks (chronic longCOVID), which affects 13% of patients for more than 4 weeks, 5% for more than 8 weeks, and 2% for more than 12 weeks [159], and is frequently observed in children and adolescents [160]. In some cases, the syndrome is referred to the presence of symptoms in patients who have passed the acute phase of the disease, being negative to the common tests for SARS CoV-2 diagnosis [161].
As regards the pathogenesis of the longCOVID symptoms, different hypotheses, not necessarily alternatives to each other, have been formulated: (i) survival of the virus in tissues following a sort of balance between the virus pathogenicity and the hosts immune response (in this case the pathogenesis of the neuropsychiatric symptoms would be due to direct mechanisms); (ii) triggering of delayed mechanisms based on immunopathological mechanisms (indirect mechanisms); (iii) sequelae of lesions occurring during the acute phase of the disease (postCOVID) [162].
The longCOVID symptoms frequently include neuropsychiatric symptoms, which, similarly to the symptoms of neuroCOVID, can be divided into symptoms of central origin and symptoms of peripheral origin.
The main symptoms of central origin occur with the following relative frequency: asthenia (85%); chronic fatigue with psychophysical impairment (81%); headache (68%); sensory disorders (paresthesia) (60%); taste and olfaction disorders (55%); diffuse arthralgias and myalgias (55%); depression or anxiety (47%); visual, auditory and vestibular disturbances (29-47%); and insomnia (33%) [163]. Rather characteristic is the difficulty of concentration, associated with state of mental confusion and memory disturbances, perceived by the patient as a sensation of ‘brain fog’ [164,165]. Although the longCOVID neuropsychiatric symptoms are, at least partly, the same present during the acute phase of the disease, and presumably share the pathogenic mechanisms, they tend to be more nuanced with a continuous or remitting trend [166-168].
Precise data on the pathogenesis of longCOVID symptoms and on the neuropathology on the CNS tissues after SARS CoV-2 infection are lacking. According to current views, the neuropsychiatric symptoms of longCOVID tend to resolve spontaneously, a sign that SARS CoV-2 by itself would not be able to produce chronic inflammation of the nervous tissues [165,169]. Consequently, the neuropsychiatric symptoms of the longCOVID would be related to functional disorders of the nervous system rather than to structured lesions of it. Accordingly, some neuropsychiatric symptoms of the longCOVID (e.g., headache, arthralgias and myalgias, anxiety and depression disorders, sleep disturbances, brain fog) have suggested the existence of a morbid association between the neuroCOVID and the still little known central sensitivity syndromes, including the Primary Fibromyalgia Syndrome (PFS) and the Chronic Fatigue Syndrome (CFS). This association could be linked to common pathogenetic mechanisms consisting of abnormal neuroimmunoendocrinological responses triggered by the viral infection [170,171]. The term ‘fibroCOVID’ has been coined to indicate the association between fibromyalgia and longCOVID [167]. Moreover, the affective symptoms of the longCOVID have been at least partly related with the trauma caused by the disease itself, which causes an abnormally prolonged stress reaction resulting in a Post-traumatic Stress Disorder (PTSD) [171]. In this regard, a remarkable similarity between the clinical picture of the longCOVID and that of the chronic stress syndrome has been noticed. In fact, the two syndromes share a series of mental disorders, including cognitive symptoms (sense of mental emptiness, sense of alarm, chronic fatigue, induction of negative thoughts), affective symptoms (anxiety, depression and emotion disorders), behavioral symptoms (interpersonal problems, tendency to self-isolation), somatic symptoms (muscle tension, tremor), and visceral symptoms affecting respiratory system (tachypnea, shortness of breath), cardiovascular system (tachycardia, palpitations, chest pain), digestive symptoms (nausea, inappetence, alteration of intestinal transit, abdominal pain) and integumentary system (skin rash, profuse sweating) [172]. In contrast, Diffusion Tensor Imaging (DTI) and 3D high-resolution T1WI sequences acquired in recovered COVID-19 have revealed possible disruption to microstructural and functional integrity of brain tissues suggesting the long-term consequences of SARS-CoV-2 [173]. Finally, it is important to point out that the affective disturbances may in some cases be attributed to the changes of lifestyle and of interpersonal relationships and to the prolonged loneliness condition that COVID-19 patients underwent during the disease [174].
The symptoms of peripheral origin mainly occur with the late onset of the GBSS following SARS CoV-2 infection [175,176].
The appearance of a neuropsychiatric syndrome in the course of COVID-19 has been described in an extremely variable percentage of cases (from 36 to 60%) [6-9], probably because the different countries have adopted non-homogeneous strategies in the collection of epidemiological data [10].
The neuroCOVID symptoms may be due to neuropathological lesions caused by direct or indirect damaging effect of SARS CoV-2 on the nervous tissues (Figure 1). In the first case, the virus is usually detectable in the nervous tissues, while in the second case, this detection and therefore the pathogenic role is rarely possible [177].
Actually, the above division into direct and indirect mechanisms is not easy to apply since the different symptoms of neuroCOVID, due to the considerable frequency of the variables, continuously change their clinical expression. An element that notably contributes to the neuroCOVID symptoms variability is linked to the fact that the different neuropathogenic mechanisms can variously combine, coexisting or overlapping each other, and mutually influence their clinical manifestation and give rise to extremely complex neuropsychiatric syndromes different from patient to patient [29]. The possibility of these interactions must be maximally taken into consideration during the management of COVID-19 patients, because they can trigger a vicious circle that causes a rapid aggravation of the clinical picture and aggravates the prognosis of COVID-19 (Figure 2).
Figure 2: Examples of interactions among neuropathogenic mechanisms in the course of COVID-19, responsible for vicious circles that inevitably lead to a serious worsening of neuroCOVID symptoms.
Examples of these interactions have been provided below. (i) Focal encephalitis localized in the olfactory regions or in the medulla oblongata tegmentum (direct mechanism) can easily spread to neighboring regions, for example to the hypothalamus or to the reticular formation, where it involves regulatory centers of the respiratory and/or cardiovascular systems; this causes the onset or the worsening of the respiratory and circulatory disorders, which in turn have a negative impact on the nervous tissues, causing a DHE (indirect mechanism) (Figure 2a). (ii) Focal encephalitis localized in hypothalamus (direct mechanism) can affect hypothalamic nuclei involved in the regulation of the immune system favoring the onset of a cytokine-release syndrome which in turn can lead to a DCRE (indirect mechanism) (Figure 2b). (iii) DCRE (indirect mechanism) can cause inflammatory processes that affect the encephalic vessels, causing morphofunctional alterations of the BBB and subsequent massive invasion of nervous tissues by the virus through the hematogenous route (direct mechanism) (Figure 2c). (iv) Disorders of hemocoagulation determined by SARS CoV-2 (direct/indirect mechanism) can cause thromboembolic phenomena that affect cerebral blood vessels, causing cerebral infarction (indirect mechanism). (v) Focal or diffuse encephalitis (direct/indirect mechanism) can cause changes of nervous tissue antigenicity responsible for abnormal immune system responses supported by mechanisms of molecular mimicry or of bystander activation. In reality, the series of examples could still be very long.
All these conditions represent a serious risk for the patient’s survival. The onset of neuropsychiatric symptoms in a COVID-19 patient is a fearful event that must be promptly recognized and adequately treated: a COVID-19 patient must always be subjected to the most accurate clinical and instrumental examinations for early recognition of neuropsychiatric symptoms and subsequent management of them [178].
Also the follow-up study in order to ascertain the long-term consequences of neuroCOVID must be carried out with accuracy: the neuropsychiatric symptoms of longCOVID, although milder than those present in the acute phase of the disease, can lead to significant disability and deeply impact on the quality of life of patients. To date, unfortunately, there are no specific therapies to treat disorders related to the longCOVID. Patients are forced to live with the symptoms until their regression and try, as far as possible, to alleviate them, finding personalized solutions that can include: drug therapy for the treatment of pain syndromes, physical rehabilitation exercises, diets aimed at restoring optimal conditions of weight and muscle mass, and, finally, psychological support for those with PTSD.
In these two years of the COVID-19 pandemic, many data on the pathogenetic mechanisms of neuroCOVID symptoms are still poorly known and the current opinions on them are often conflicting. Future research is needed in order to clarify the pathogenic mechanisms of all the neuroCOVID symptoms and take advantage of these to implement a series of measures against SARS CoV-2: (i) to standardize the collection of clinical data, in order to specify the origin of the neuroCOVID symptoms, evaluate their evolutionary trend and obtain precise data on their epidemiology; (ii) to deserve a multidisciplinary approach to treatment of the disease, in a perspective that considers COVID-19 as a multisystem disease; (iii) to subject the highest possible number of patients died with neuroCOVID to autopsy, in order to verify the localization and the type of neuropathological lesions, verify the presence of SARS CoV-2 markers in nervous lesions, and understand as much as possible the pathophysiological mechanisms that led to the patient’s death; (iv) to implement research programs aimed at identifying predisposing factors to the onset of neuroCOVID symptoms; (v) to activate experimental research programs on animal models, human neural cells and brain organoids aimed at investigating the possible neuropathogenic mechanisms through which SARS CoV-2 can affect the nervous system in order to counter or prevent them.
Finally, the appearance of variants of the wild type of SARS CoV-2 (Alpha variant, UK, Sep-2020; Beta variant, South Africa, May-2020; Gamma, Brazil, Nov-2020; Delta, Oct-2020; and Omicron, multiple countries, Nov-2021) has raised the question of whether these variants exhibit changes in neuropathogenicity. The available data on this important topic are still scarce and it is necessary that experimental and clinical studies on it are activated as soon as possible.
The work was supported by grants from the University of Bari to Vincenzo Benagiano (Ricerca Scientifica Ateneo-U.P.B. Benagiano 006188).
None.
This work is dedicated to countless patients scattered around the world, which paid with their own life the tribute for the progress of scientific research. To them goes the profound gratitude of the authors.
Conceptualization: AR, VB; Data curation, Formal analysis: AR, SZ, GI, VC, MS, VB; Writing-original draft: AR, VB; Writing-review & editing: AR, SZ, GI, VC, MS, VB.
- Guan WJ, Ni ZY, Hu Y, Liang W-H, Ou CQ, et al. (2020) Clinical characteristics of Coronavirus Disease 2019 in China. N Engl J Med 382: 1708-1720. [Ref.]
- Huang C, Wang Y, Li X, Ren L, Zhao J, et al. (2020) Clinical features of patients infected with 2019 novel Coronavirus in Wuhan, China. Lancet 395: 497-506. [Ref.]
- Korber B, Fischer WM, Gnanakaran S, Yoon H, Theiler J, et al. (2020) Tracking changes in SARS-CoV-2 spike: evidence that D614G increases infectivity of the COVID-19 virus. Cell 182: 812-827. [Ref.]
- Boehm E, Kronig I, Neher RA, Eckerle I, Vetter P, et al. (2021) Novel SARS-CoV-2 variants: the pandemics within the pandemic. Clin Microbiol Infect 27: 1109-1117. [Ref.]
- Shahhosseini N, Babuadze GG, Wong G, Kobinger GP (2021) Mutation signatures and in silico docking of novel SARS-CoV-2 variants of concern. Microorganisms 9: 926.
- Helbok R, Chou SH, Beghi E, Mainali S, Frontera J, et al. (2020) NeuroCOVID: it’s time to join forces globally. Lancet Neurol 19: 805- 806. [Ref.]
- Chou SH, Beghi E, Helbok R, Moro E, Sampson J, et al. (2021) Global Incidence of Neurological Manifestations Among Patients Hospitalized With COVID-19-A Report for the GCS-NeuroCOVID Consortium and the ENERGY Consortium. JAMA Netw Open 4: e2112131. [Ref.]
- Helms J, Kremer S, Merdji H, Clere-Jehl R, Schenck M, et al. (2020) Neurologic features in severe SARS-CoV-2 infection. N Engl J Med 382: 2268-2270. [Ref.]
- Mao L, Jin H, Wang M, Hu Y, Chen S, et al. (2020) Neurologic manifestations of hospitalized patients with Coronavirus Disease 2019 in Wuhan, China. JAMA Neurol 77: 683-690. [Ref.]
- Beghi E, Michael BD, Solomon T, Westenberg E, Winkler AS, et al. (2021) Approaches to understanding COVID-19 and its neurological associations. Ann Neurol 89: 1059-1067. [Ref.]
- Pascarella G, Strumia A, Piliego C, Bruno F, Del Buono R, et al. (2020) COVID-19 diagnosis and management: a comprehensive review. J Intern Med 288: 192-206. [Ref.]
- Pollard CA, Morran MP, Nestor-Kalinoski AL (2020) The COVID-19 pandemic: a global health crisis. Physiol Genomics 52: 549-557. [Ref.]
- Mirfazeli FS, Sarabi-Jamab A, Jahanbakhshi A, Kordi A, Javadnia P, et al. (2020) Neuropsychiatric manifestations of COVID-19 can be clustered in three distinct symptom categories. Sci Rep 10: 20957. [Ref.]
- Herman C, Mayer K, Sarwal A (2020) Scoping review of prevalence of neurologic comorbidities in patients hospitalized for COVID-19. Neurology 95: 77-84. [Ref.]
- Kumar M, Thakur AK (2020) Neurological manifestations and comorbidity associated with COVID-19: an overview. Neurol Sci 41: 3409-3418. [Ref.]
- Xie J, Tong Z, Guan X, Du B, Qiu H (2020) Clinical characteristics of patients who died of coronavirus disease 2019 in China. JAMA Netw Open 3: e205619. [Ref.]
- Kumar A, Prasoon P, Kumari C, Pareek V, Faiq MA, et al. (2021) SARSCoV- 2-specific virulence factors in COVID-19. J Med Virol 93: 1343- 1350. [Ref.]
- Lou JJ, Movassaghi M, Gordy D, Olson MG, Zhang T, et al. (2021) Neuropathology of COVID-19 (neuro-COVID): clinicopathological update. Free Neuropathol 2: 2. [Ref.]
- Banerjee A, Pasea L, Harris S, Gonzalez-Izquierdo A, Torralbo A, et al. (2020) Estimating excess 1-year mortality associated with the COVID-19 pandemic according to underlying conditions and age: a population-based cohort study. Lancet 395: 1715-1725. [Ref.]
- Klaassen K, Stankovic B, Zukic B, Kotur N, Gasic V, et al. (2020) Functional prediction and comparative population analysis of variants in genes for proteases and innate immunity related to SARS-CoV-2 infection. Infect Genet Evol 84: 104498. [Ref.]
- Marin BG, Aghagoli G, Lavine K, Yang L, Siff EJ, et al. (2021) Predictors of COVID-19 severity: a literature review. Rev Med Virol 31: 1-10. [Ref.]
- Jesuthasan A, Massey F, Manji H, Zandi MS, Wiethoff S (2021) Emerging potential mechanisms and predispositions to the neurological manifestations of COVID-19. J Neurol Sci 428: 117608. [Ref.]
- Oliveira V, Seabra M, Rodrigues R, Carvalho V, Mendes M, et al. (2021) Neuro-COVID frequency and short-term outcome in the Northern Portuguese population. Eur J Neurol 28: 3360-3368. [Ref.]
- Ferrarese C, Silani V, Priori A, Galimberti S, Agostoni E, et al. (2020) An Italian multicenter retrospective-prospective observational study on neurological manifestations of COVID-19 (NEUROCOVID). Neurol Sci 41: 1355-1359. [Ref.]
- Frontera J, Mainali S, Fink EL, Robertson CL, Schober M, et al. (2020) Global Consortium Study of Neurological Dysfunction in COVID-19 (GCS-NeuroCOVID): Study Design and Rationale. Neurocrit Care 33: 25-34. [Ref.]
- Guerrero JI, Barragan LA., Martinez JD, Montoya JP, Pena A, et al. (2021) Central and peripheral nervous system involvement by COVID-19: a systematic review of the pathophysiology, clinical manifestations, neuropathology, neuroimaging, electrophysiology, and cerebrospinal fluid findings. BMC Infect Dis 21: 515. [Ref.]
- Ahmed MU, Hanif M, Ali MJ, Haider MA, Kherani D, et al. (2020) Neurological Manifestations of COVID-19 (SARS-CoV-2): A Review. Front Neurol 11: 518. [Ref.]
- Ellul MA, Benjamin M, Singh B, Lant S, Michael BD, et al. (2020) Neurological associations of COVID-19. Lancet Neurol 19: 767-783. [Ref.]
- Leven Y, Bosel J (2021) Neurological manifestations of COVID-19 - an approach to categories of pathology. Neurol Res Pract 3: 39. [Ref.]
- Carsana L, Sonzogni A, Nasr A, Rossi RS, Pellegrinelli A, et al. (2020) Pulmonary post-mortem findings in a series of COVID-19 cases from northern Italy: a two-centre descriptive study. Lancet Infect Dis 20: 1135-1140. [Ref.]
- Wang D, Hu B, Hu C, Zhu F, Liu X, et al. (2020) Clinical Characteristics of 138 Hospitalized Patients With 2019 Novel Coronavirus-Infected Pneumonia in Wuhan, China. JAMA 323: 1061-1069. [Ref.]
- Wu F, Zhao S, Yu B, Chen Y-M, Wang W, et al. (2020) A new Coronavirus associated with human respiratory disease in China. Nature 579: 265-269. [Ref.]
- Gu J, Han B, Wang J (2020) COVID-19: Gastrointestinal Manifestations and Potential Fecal-Oral Transmission. Gastroenterology 158: 1518- 1519.[Ref.]
- Atukorallaya DS, Ratnayake RK (2021) Oral Mucosa, Saliva, and COVID-19 Infection in Oral Health Care. Front Med 8: 656926. [Ref.]
- Dockery DM, Rowe SG, Murphy MA, Krzystolik MG (2020) The Ocular Manifestations and Transmission of COVID-19: Recommendations for Prevention. J Emerg Med 59: 137-140. [Ref.]
- Zhao Q, Fang X, Pang Z, Zhang B, Liu H, et al. (2020) COVID-19 and cutaneous manifestations: a systematic review. J Eur Acad Dermatol Venereol 34: 2505-2510. [Ref.]
- Bourgonje AR, Abdulle AE, Timens W, Hillebrands J-L, Navis GJ, et al. (2020) Angiotensin-converting enzyme 2 (ACE2), SARS-CoV-2 and the pathophysiology of coronavirus disease 2019 (COVID-19). J Pathol 251: 228-248. [Ref.]
- Yang J, Petitjean SJL, Koehler M, Zhang Q, Dumitru AC, et al. (2020) Molecular interaction and inhibition of SARS-CoV-2 binding to the ACE2 receptor. Nat Commun 11: 4541. [Ref.]
- Hamming I, Timens W, Bulthuis MLC, Lely AT, Navis G, et al. (2004) Tissue distribution of ACE2 protein, the functional receptor for SARS Coronavirus. A first step in understanding SARS pathogenesis. J Pathol 203: 631-637. [Ref.]
- Guney C, Akar F (2021) Epithelial and endothelial expressions of ACE2: SARS-CoV-2 entry routes. J Pharm Pharm Sci 24: 84-93. [Ref.]
- Valyaeva AA, Zharikova AA, Kasianov AS, Vassetzky YS, Sheval EV (2020) Expression of SARS-CoV-2 entry factors in lung epithelial stem cells and its potential implications for COVID-19. Sci Rep 10: 17772. [Ref.]
- Ratajczak MZ, Bujko K, Ciechanowicz A, Sielatycka K, Cymer M, et al. (2021) SARS-CoV-2 Entry Receptor ACE2 Is Expressed on Very Small CD45-Precursors of Hematopoietic and Endothelial Cells and in Response to Virus Spike Protein Activates the Nlrp3 Inflammasome. Stem Cell Rev Rep 17: 266-277. [Ref.]
- Kuba K, Imai Y, Rao S, Gao H, Guo F, et al. (2005) A crucial role of angiotensin converting enzyme 2 (ACE2) in SARS Coronavirusinduced lung injury. Nat Med 11: 875-879. [Ref.]
- Hui KPY, Cheung M-C, Perera RAPM, Ng K-C, Bui CHT, et al. (2020) Tropism, replication competence, and innate immune responses of the coronavirus SARS-CoV-2 in human respiratory tract and conjunctiva: an analysis in ex-vivo and in-vitro cultures. Lancet Respir Med 8: 687-695. [Ref.]
- Xu H, Zhong L, Deng J, Peng J, Dan H, et al. (2020) High expression of ACE2 receptor of 2019-nCoV on the epithelial cells of oral mucosa. Int J Oral Sci 12: 8. [Ref.]
- Zou X, Chen K, Zou J, Han P, Hao J, et al. (2020) Single-cell RNA-seq data analysis on the receptor ACE2 expression reveals the potential risk of different human organs vulnerable to 2019-nCoV infection. Front Med 14: 185-192. [Ref.]
- Lange C, Wolf J, Auw-Haedrich C, Schlecht A, Boneva S, et al. (2020) Expression of the COVID-19 receptor ACE2 in the human conjunctiva. J Med Virol 92: 2081-2086. [Ref.]
- Grzegrzolka J, Swiatko K, Pula B, Zamirska A, Olbromski M, et al. (2013) ACE and ACE2 expression in normal and malignant skin lesions. Folia Histochem Cytobiol 51: 232-238. [Ref.]
- Baig AM, Khaleeq A, Ali U, Syeda H (2020) Evidence of the COVID‐19 virus targeting the CNS: tissue distribution, host‐virus interaction, and proposed neurotropic mechanisms. ACS Chem Neurosci 11: 995-998. [Ref.]
- Jakhmola S, Indari O, Chatterjee S, Jha HC (2020) SARS-CoV-2, an underestimated pathogen of the nervous system. SN Compr Clin Med. [Ref.]
- Kanberg N, Ashton NJ, Andersson L-M, Yilmaz A, Lindh M, et al. (2020) Neurochemical evidence of astrocytic and neuronal injury commonly found in COVID-19. Neurology 95: e1754-e1759. [Ref.]
- Xu J, Lazartigues E (2020) Expression of ACE2 in human neurons supports the neuro-invasive potential of COVID-19 virus. Cell Mol Neurobiol 42: 305-309. [Ref.]
- Bodnar B, Patel K, Ho W, Luo JJ, Hu W (2021) Cellular mechanisms underlying neurological/neuropsychiatric manifestations of COVID-19. J Med Virol 93: 1983-1998. [Ref.]
- Chen R, Wang K, Yu J, Howard D, French L, et al. (2021) The Spatial and Cell-Type Distribution of SARS-CoV-2 Receptor ACE2 in the Human and Mouse Brains. Front Neurol 11: 573095. [Ref.]
- McKinley MJ, Albiston AL, Allen AM, Mathai ML, May CN, et al. (2003) The brain renin-angiotensin system: location and physiological roles. Int J Biochem Cell Biol 35: 901-918. [Ref.]
- Mohammed M, Berdasco C, Lazartigues E (2020) Brain angiotensin converting enzyme-2 in central cardiovascular regulation. Clin Sci 134: 2535-2547. [Ref.]
- Song E, Zhang C, Israelow B, Lu-Culligan A, Prado AV, et al. (2021) Neuroinvasion of SARS-CoV-2 in human and mouse brain. J Exp Med 218: e20202135. [Ref.]
- Matschke J, Lutgehetmann M, Hagel C, Sperhake JP, Schroder AS, et al. (2020) Neuropathology of patients with COVID-19 in Germany: a post-mortem case series. Lancet Neurol 19: 919-929. [Ref.]
- Netland J, Meyerholz DK, Moore S, Cassell M, Perlman S (2008) Severe acute respiratory syndrome coronavirus infection causes neuronal death in the absence of encephalitis in mice transgenic for human ACE2. J Virol 82: 7264-7275.
- Li K, Wohlford-Lenane C, Perlman S, Zhao J, Jewell AK, et al. (2016) Middle East Respiratory Syndrome Coronavirus Causes Multiple Organ Damage and Lethal Disease in Mice Transgenic for Human Dipeptidyl Peptidase 4. J Infect Dis 213: 712-722. [Ref.]
- Koyuncu OO, Hogue IB, Enquist LW (2013) Virus infections in the nervous system. Cell Host Microbe 13: 379-393. [Ref.]
- Dube M, Le Coupanec A, Wong AHM, Rini JM, Desforges M, et al. (2018) Axonal transport enables neuron-to-neuron propagation of human Coronavirus OC43. J Virol 92: e00404-e00418. [Ref.]
- Wu Y, Xu X, Chen Z, Duan J, Hashimoto K, et al. (2020) Nervous system involvement after infection with COVID-19 and other Coronaviruses. Brain Behav Immun 87: 18-22. [Ref.]
- Espindola OM, Brandao CO, Gomes YCP, Siqueira M, Soares CN, et al. (2021) Cerebrospinal fluid findings in neurological diseases associated with COVID-19 and insights into mechanisms of disease development. Int J Infect Dis 102: 155-162. [Ref.]
- Huang YH, Jiang D, Huang JT (2020) SARS-CoV-2 detected in cerebrospinal fluid by PCR in a case of COVID-19 encephalitis. Brain Behav Immun 87: 149. [Ref.]
- Liu JM, Tan B-H, Wu S, Gui Y, Suo JL, et al. (2021) Evidence of central nervous system infection and neuroinvasive routes, as well as neurological involvement, in the lethality of SARS-CoV-2 infection. J Med Virol 93: 1304-1313. [Ref.]
- Brann DH, Tsukahara T, Weinreb C, Lipovsek M, den Berge KV, et al. (2020) Non-neuronal expression of SARS-CoV-2 entry genes in the olfactory system suggests mechanisms underlying COVID-19- associated anosmia. Sci Adv 6: eabc5801. [Ref.]
- Chen M, Shen W, Rowan NR, Kulaga H, Hillel A, et al. (2020) Elevated ACE-2 expression in the olfactory neuroepithelium: implications for anosmia and upper respiratory SARS-CoV-2 entry and replication. Eur Respir J 56: 2001948. [Ref.]
- Lempriere S (2021) SARS-CoV-2 detected in olfactory neurons. Nat Rev Neurol 17: 63. [Ref.]
- Meinhardt J, Radke J, Dittmayer C, Franz J, Thomas C, et al. (2021) Olfactory transmucosal SARS-CoV-2 invasion as a port of central nervous system entry in individuals with COVID-19. Nat Neurosci 24: 168-175.
- Serrano GE, Walker JE, Arce R, Glass MJ, Vargas D, et al. (2021) Mapping of SARS-CoV-2 brain invasion and histopathology in COVID-19 disease. medRxiv. [Ref.]
- Morbini P, Benazzo M, Verga L, Pagella FG, Mojoli F, et al. (2020) Ultrastructural evidence of direct viral damage to the olfactory complex in patients testing positive for SARS-CoV-2. JAMA Otolaryngol Head Neck Surg 146: 972-973. [Ref.]
- Kumar A, Pareek V, Prasoon P, Faiq MA, Kumar P, et al. (2020) Possible routes of SARS-CoV-2 invasion in brain: In context of neurological symptoms in COVID-19 patients. J Neurosci Res 98: 2376-2383.
- Li YC, Bai W-Z, Hashikawa T (2020) The neuroinvasive potential of SARS-CoV2 may play a role in the respiratory failure of COVID-19 patients. J Med Virol 92: 552-555.
- Imboden H, Patil J, Nussberger J, Nicoud F, Hess B, et al. (2009) Endogenous angiotensinergic system in neurons of rat and human trigeminal ganglia. Regul Pept 154: 23-31. [Ref.]
- Shiers S, Ray PR, Wangzhou A, Sankaranarayanan I, Tatsui CE, et al. (2020) ACE2 and SCARF expression in human dorsal root ganglion nociceptors: implications for SARS-CoV-2 virus neurological effects. Pain 161: 2494-2501. [Ref.]
- Chow CCN, Magnussen J, Ip J, Su Y (2020) Acute transverse myelitis in COVID-19 infection. BMJ Case Rep 13: e236720. [Ref.]
- Zhao K, Huang J, Dai D, Feng Y, Liu L, et al. (2020) Acute myelitis after SARS-CoV-2 infection: a case report. medRxiv. [Ref.]
- Berger JR (2020) COVID-19 and the nervous system. J Neurovirol 26: 143-148. [Ref.]
- Zhou Z, Kang H, Li S, Zhao X (2020) Understanding the neurotropic characteristics of SARS-CoV-2: from neurological manifestations of COVID-19 to potential neurotropic mechanisms. J Neurol 267: 2179- 2184. [Ref.]
- Li Y, Schneider A, Mehta A, Sade-Feldman M, Kays KR, et al. (2021) SARS-CoV-2 viremia is associated with distinct proteomic pathways and predicts COVID-19 outcomes. J Clin Invest 131: e148635. [Ref.]
- Achar A, Ghosh C (2020) COVID-19-associated neurological disorders: the potential route of CNS invasion and blood-brain relevance. Cells 9: 2360. [Ref.]
- Wang W, Xu Y, Gao R, Lu R, Han K, et al. (2020) Detection of SARSCoV- 2 in different types of clinical specimens. JAMA 323: 1843-1844. [Ref.]
- Zheng S, Fan J, Yu F, Feng B, Lou B, et al. (2020) Viral load dynamics and disease severity in patients infected with SARS-CoV-2 in Zhejiang province, China, January-March 2020: retrospective cohort study. BMJ 369: m1443. [Ref.]
- Buzhdygan TP, DeOre BJ, Baldwin-Leclair A, Bullock TA, McGary HM, et al. (2020) The SARS-CoV-2 spike protein alters barrier function in 2D static and 3D microfluidic in-vitro models of the human bloodbrain barrier. Neurobiol Dis 146: 105131. [Ref.]
- Kantonen J, Mahzabin S, Mayranpaa MI, Tynninen O, Paetau A, et al. (2020) Neuropathologic features of four autopsied COVID-19 patients. Brain Pathol 30: 1012-1016. [Ref.]
- Solomon IH, Normandin E, Bhattacharyya S, Mukerji SS, Keller K, et al. (2020) Neuropathological features of Covid-19. N Engl J Med 383: 989-992. [Ref.]
- Ghajarzadeh M, Bonavita S (2020) Are patients with multiple sclerosis (MS) at higher risk of COVID-19 infection? Neurol Sci 41: 2315-2316. [Ref.]
- Matias-Guiu JA, Pytel V, Matias-Guiu J (2020) Death Rate Due to COVID-19 in Alzheimer’s Disease and Frontotemporal Dementia. J Alzheimers Dis 78: 537-541. [Ref.]
- Huang S, Wang J, Liu F, Liu J, Cao G, et al. (2020) COVID-19 patients with hypertension have more severe disease: a multicenter retrospective observational study. Hypertens Res 43: 824-831. [Ref.]
- Xu K, Cai H, Shen Y, Ni Q, Chen Y, et al. (2020) Management of Corona Virus Disease-19 (COVID-19): the Zhejiang experience. Zhejiang Da Xue Xue Bao Yi Xue Ban 49: 147-157. [Ref.]
- Berlin DA, Gulick RM, Martinez FJ (2020) Severe Covid-19. N Engl J Med 383: 2451-2460. [Ref.]
- Bikdeli B, Madhavan MV, Jimenez D, Chuich T, Dreyfus I, et al. (2020) COVID-19 and Thrombotic or Thromboembolic Disease: Implications for Prevention, Antithrombotic Therapy, and Follow-Up: JACC Stateof- the-Art Review. J Am Coll Cardiol 75: 2950-2973. [Ref.]
- Varga Z, Flammer AJ, Steiger P, Haberecker M, Andermatt R, et al. (2020) Endothelial cell infection and endotheliitis in COVID19. Lancet 395: 1417-1418. [Ref.]
- Wichmann D, Sperhake J-P, Lutgehetmann M, Steurer S, Edler C, et al. (2020) Autopsy Findings and Venous Thromboembolism in Patients With COVID-19: A Prospective Cohort Study. Ann Intern Med 173: 268-277. [Ref.]
- Tajbakhsh A, Hayat SMG, Taghizadeh H, Akbari A, Inabadi M, et al. (2021) COVID-19 and cardiac injury: clinical manifestations, biomarkers, mechanisms, diagnosis, treatment, and follow up. Expert Rev Anti Infect Ther 19: 345-357. [Ref.]
- Reichard RR, Kashani KB, Boire NA, Constantopoulos E, Guo Y, et al. (2020) Neuropathology of COVID-19: a spectrum of vascular and Acute Disseminated Encephalomyelitis (ADEM)-like pathology. Acta Neuropathol 140: 1-6. [Ref.]
- Kremer S, Lersy F, de Seze J, Ferré J-C, Maamar A, et al. (2020) Brain MRI Findings in Severe COVID-19: A Retrospective Observational Study. Radiology 297: E242-E251. [Ref.]
- Politi LS, Salsano E, Grimaldi M (2020) Magnetic Resonance Imaging Alteration of the Brain in a Patient With Coronavirus Disease 2019 (COVID-19) and Anosmia. JAMA Neurol 77: 1028-1029. [Ref.]
- Li Y, Li M, Wang M, Zhou Y, Chang J, et al. (2020) Acute cerebrovascular disease following COVID-19: a single center, retrospective, observational study. Stroke Vasc Neurol 5: 279-284. [Ref.]
- Patel SD, Kollar R, Troy P, Song X, Khaled M, et al. (2020) Malignant Cerebral Ischemia in A COVID-19 Infected Patient: Case Review and Histopathological Findings. J Stroke Cerebrovasc Dis 29: 105231. [Ref.]
- Shahjouei S, Naderi S, Li J, Khan A, Chaudhary D, et al. (2020) Risk of stroke in hospitalized SARS-CoV-2 infected patients: a multinational study. EBioMedicine 59: 102939. [Ref.]
- Kishfy L, Casasola M, Banankhah P, Parvez A, Jan YJ, et al. (2020) Posterior Reversible Encephalopathy Syndrome (PRES) as a neurological association in severe Covid-19. J Neurol Sci 414: 116943. [Ref.]
- Parauda SC, Gao V, Gewirtz AN, Parikh NS, Merkler AE, et al. (2020) Posterior reversible encephalopathy syndrome in patients with COVID-19. J Neurol Sci 416: 117019. [Ref.]
- Merad M, Martin JC (2020) Pathological inflammation in patients with COVID-19: a key role for monocytes and macrophages. Nature Rev Immunol 20: 355-362. [Ref.]
- Hu B, Huang S, Yin L (2021) The cytokine storm and COVID-19. J Med Virol 93: 250-256. [Ref.]
- Qin C, Zhou L, Hu Z, Zhang S, Yang S, et al. (2020) Dysregulation of immune response in patients with Coronavirus 2019 (COVID-19) in Wuhan, China. Clin Infect Dis 71: 762-768. [Ref.]
- Sankowski R, Mader S, Valdes-Ferrer SI (2015) Systemic inflammation and the brain: novel roles of genetic, molecular, and environmental cues as drivers of neurodegeneration. Front Cell Neurosci 9: 28. [Ref.]
- Ye Q, Wang B, Mao J (2020) The pathogenesis and treatment of the ‘Cytokine Storm’ in COVID-19. J Infect 80: 607-613. [Ref.]
- Kumar N, Kumar S, Kumar A, Pati BK, Kumar A, et al. (2020) Acute Necrotizing Encephalitis as a Probable Association of COVID-19. Indian J Crit Care Med 24: 991-994. [Ref.]
- Perrin P, Collongues N, Baloglu S, Bedo D, Bassand X, et al. (2021) Cytokine release syndrome-associated encephalopathy in patients with COVID-19. Eur J Neurol 28: 248-258. [Ref.]
- Akca UK, Kesici S, Ozsurekci Y, Aykan HH, Batu ED, et al. (2020) Kawasaki-like disease in children with COVID-19. Rheumatol Int 40: 2105-2115. [Ref.]
- Jones VG, Mills M, Suarez D, Hogan CA, Yeh D, et al. (2020) COVID-19 and Kawasaki disease: novel virus and novel case. Hosp Pediatr 10: 537-540. [Ref.]
- Hanff TC, Mohareb AM, Giri J, Cohen JB, Chirinos JA (2020) Thrombosis in COVID-19. Am J Hematol 95: 1578-1589. [Ref.]
- Iba T, Levy JH, Levi M, Thachil J (2020) Coagulopathy in COVID-19. J Thromb Haemost 18: 2103-2109. [Ref.]
- Salle V (2021) Coronavirus-induced autoimmunity. Clin Immunol 226: 108694. [Ref.]
- Boucher A, Desforges M, Duquette P, Talbot PJ (2007) Long-term human coronavirus-myelin cross-reactive T-cell clones derived from multiple sclerosis patients. Clin Immunol 123: 258-267. [Ref.]
- Franke C, Ferse C, Kreye J, Reincke SM, Sanchez-Sendin E, et al. (2021) High frequency of cerebrospinal fluid autoantibodies in COVID-19 patients with neurological symptoms. Brain Behav Immun 93: 415-419. [Ref.]
- Akhoundi FH, Sahraian MA, Moghadasi AN (2020) Neuropsychiatric and cognitive effects of the COVID-19 outbreak on multiple sclerosis patients. Mult Scler Relat Disord 41: 102164. [Ref.]
- Suh J, Mukerji SS, Collens SI, Padera RF Jr, Pinkus GS, et al. (2021) Skeletal Muscle and Peripheral Nerve Histopathology in COVID-19. Neurology 97: e849-e858. [Ref.]
- Dalakas MC (2020) Guillain-Barré syndrome: the first documented COVID-19-triggered autoimmune neurologic disease: more to come with myositis in the offing. Neurol Neuroimmunol Neuroinflamm 7: e781. [Ref.]
- Lucchese G, Floel A (2020) SARS-CoV-2 and Guillain-Barré syndrome: molecular mimicry with human heat shock proteins as potential pathogenic mechanism. Cell Stress Chaperones 25: 731-735. [Ref.]
- Dufour C, Co T-K, Liu A (2021) GM1 ganglioside antibody and COVID-19 related Guillain Barre syndrome-A case report, systemic review and implication for vaccine development. Brain Behav Immun Health 12: 100203. [Ref.]
- Filosto M, Piccinelli SC, Gazzina S, Foresti C, Frigeni B, et al. (2021) Guillain-Barré syndrome and COVID-19: an observational multicentre study from two Italian hotspot regions. J Neurol Neurosurg Psychiatry 92: 751-756. [Ref.]
- Keddie S, Pakpoor J, Mousele C, Pipis M, Machado PM, et al. (2021) Epidemiological and cohort study finds no association between COVID-19 and Guillain-Barré syndrome. Brain 144: 682-693. [Ref.]
- Lunn MP, Cornblath DR, Jacobs BC, Querol L, van Doorn PA, et al. (2021) COVID-19 vaccine and Guillain-Barré syndrome: let’s not leap to associations. Brain 144: 357-360. [Ref.]
- Agyeman AA, Chin KL, Landersdorfer CB, Liew D, Ofori-Asenso R (2020) Smell and Taste Dysfunction in Patients With COVID-19: A Systematic Review and Meta-analysis. Mayo Clin Proc 95: 1621- 1631. [Ref.]
- Meng X, Deng Y, Dai Z, Meng Z (2020) COVID-19 and anosmia: A review based on up-to-date knowledge. Am J Otolaryngol 41: 102581. [Ref.]
- Vaira LA, Salzano G, Fois AG, Piombino P, De Riu G (2020) Potential pathogenesis of ageusia and anosmia in COVID-19 patients. Int Forum Allergy Rhinol 10: 1103-1104. [Ref.]
- Ciorba A, Hatzopoulos S, Cogliandolo C, Bianchini C, Renna M, et al. (2020) Functional Magnetic Resonance Imaging in the Olfactory Perception of the Same Stimuli. Life 11: 11. [Ref.]
- Yousefi-Koma A, Haseli S, Bakhshayeshkaram M, Raad N, Karimi- Galougahi M (2021) Multimodality Imaging With PET/CT and MRI Reveals Hypometabolism in Tertiary Olfactory Cortex in Parosmia of COVID-19. Acad Radiol 28: 749-751. [Ref.]
- Chigr F, Merzouki M, Najimi M (2020) Autonomic brain centers and pathophysiology of COVID-19. ACS Chem Neurosci 11: 1520-1522. [Ref.]
- D’Amico F, Baumgart DC, Danese S, Peyrin‐Biroulet L (2020) Diarrhea during COVID‐19 infection: pathogenesis, epidemiology, prevention and management. Clin Gastroenterol Hepatol 18: 1663-1672. [Ref.]
- Manganelli F, Vargas M, Iovino A, Iacovazzo C, Santoro L, et al. (2020) Brainstem involvement and respiratory failure in COVID-19. Neurol Sci 41: 1663-1665. [Ref.]
- Shi S, Qin M, Shen B, Cai Y, Liu T, et al. (2020) Association of Cardiac Injury With Mortality in Hospitalized Patients With COVID-19 in Wuhan, China. JAMA Cardiol 5: 802-810. [Ref.]
- Andrews PLR, Cai W, Rudd JA, Sanger GJ (2021) COVID‐19, nausea, and vomiting. J Gastroenterol Hepatol 36: 646-656. [Ref.]
- Saniasiaya J, Islam MA, Abdullah B (2021) Prevalence and Characteristics of Taste Disorders in Cases of COVID-19: A Metaanalysis of 29,349 Patients. Otolaryngol Head Neck Surg 165: 33-42. [Ref.]
- Georgalas C, Jovancevic L (2012) Gustatory rhinitis. Curr Opin Otolaryngol Head Neck Surg 20: 9-14. [Ref.]
- Nampoothiri S, Sauve F, Ternier G, Fernandois D, Coelho C, et al. (2020) The hypothalamus as a hub for SARS-CoV-2 brain infection and pathogenesis. bioRxiv. [Ref.]
- Dantzer R (2018) Neuroimmune interactions: from the brain to the immune system and vice versa. Physiol Rev 98: 477-504. [Ref.]
- Vabret N, Britton GJ, Gruber C, Hegde S, Kim J, et al. (2020) Immunology of COVID-19: Current State of the Science. Immunity 52: 910-941. [Ref.]
- Eshak N, Abdelnabi M, Ball S, Elgwairi E, Creed K, et al. (2020) Dysautonomia: An Overlooked Neurological Manifestation in a Critically ill COVID-19 Patient. Am J Med Sci 360: 427-429. [Ref.]
- Morin CM, Carrier J, Bastien C, Godbout R, Canadian Sleep and Circadian Network (2020) Sleep and circadian rhythm in response to the COVID-19 pandemic. Can J Public Health 111: 654-657. [Ref.]
- Serrano-Castro PJ, Estivill-Torrus G, Cabezudo-Garcia P, Reyes- Bueno JA, Petersen NC, et al. (2020) Impact of SARS-CoV-2 infection on neurodegenerative and neuropsychiatric diseases: a delayed pandemic? Neurologia 35: 245-251.[Ref.]
- Deng J, Zhou F, Hou W, Silver Z, Wong CY, et al. (2021) The prevalence of depression, anxiety, and sleep disturbances in COVID-19 patients: a meta-analysis. Ann NY Acad Sci 1486: 90-111. [Ref.]
- Poyiadji N, Shahin G, Noujaim D, Stone M, Patel S, et al. (2020) COVID- 19-associated Acute Hemorrhagic Necrotizing Encephalopathy: Imaging Features. Radiology 296: E119-E120. [Ref.]
- Huo L, Xu KL, Wang H (2021) Clinical features of SARS-CoV-2- associated encephalitis and meningitis amid COVID-19 pandemic. World J Clin Cases 9: 1058-1078. [Ref.]
- Sharma M, Lioutas V-A, Madsen T, Clark J, O’Sullivan J, et al. (2020) Decline in stroke alerts and hospitalisations during the COVID-19 pandemic. Stroke Vasc Neurol 5: 403-405. [Ref.]
- Fadakar N, Ghaemmaghami S, Masoompour SM, Yeganeh BS, Akbari A, et al. (2020) A First Case of Acute Cerebellitis Associated with Coronavirus Disease (COVID-19): a Case Report and Literature Review. Cerebellum 19: 911-914. [Ref.]
- de Melo IS, Sabino-Silva R, Cunha TM, Goulart LR, Reis WL, et al. (2021) Hydroelectrolytic Disorder in COVID-19 patients: Evidence Supporting the Involvement of Subfornical Organ and Paraventricular Nucleus of the Hypothalamus. Neurosci Biobehav Rev 124: 216-223. [Ref.]
- Moreno-Escobar MC, Feizi P, Podury S, Tandon M, Munir B, et al. (2021) Acute cerebellitis following SARS-CoV-2 infection: A case report and review of the literature. J Med Virol 27: 101002. [Ref.]
- Manto M, Dupre N, Hadjivassiliou M, Louis ED, Mitoma H, et al. (2020) Management of Patients with Cerebellar Ataxia During the COVID-19 Pandemic: Current Concerns and Future Implications. Cerebellum 19: 562-568. [Ref.]
- Benagiano V, Rizzi A, Lorusso L, Flace P, Saccia M, et al. (2018) The functional anatomy of the cerebrocerebellar circuit: A review and new concepts. J Comp Neurol 526: 769-789. [Ref.]
- Rizzi A, Saccia M, Benagiano V (2020) Is the Cerebellum Involved in the Nervous Control of the Immune System Function? Endocr Metab Immune Disord Drug Targets 20: 546-557. [Ref.]
- Kirschenbaum D, Imbach LL, Ulrich S, Rushing EJ, Keller E, et al. (2020) Inflammatory olfactory neuropathy in two patients with COVID-19. Lancet 396: 166. [Ref.]
- Toscano G, Palmerini F, Ravaglia S, Ruiz L, Invernizzi P, et al. (2020) Guillain-Barré syndrome associated with SARS-CoV-2. N Engl J Med 382: 2574-2576. [Ref.]
- Zhao H, Shen D, Zhou H, Liu J, Chen S (2020) Guillain-Barre syndrome associated with SARS-CoV-2 infection: causality or coincidence? Lancet Neurol 19: 383-384. [Ref.]
- Gutierrez-Ortiz C, Mendez-Guerrero A, Rodrigo-Rey S, Pedro-Murillo ES, Bermejo-Guerrero L, et al. (2020) Miller Fisher syndrome and polyneuritis cranialis in COVID-19. Neurology 95: e601-e605. [Ref.]
- Nalbandian A, Sehgal K, Gupta A, Madhavan MV, McGroder C, et al. (2021) Post-acute COVID-19 syndrome. Nat Med 27: 601-615. [Ref.]
- Asadi-Pooya AA, Nemati H, Shahisavandi M, Akbari A, Emami A, et al. (2021) Long COVID in children and adolescents. World J Pediatr 17: 495-499. [Ref.]
- Yong SJ (2021) Long COVID or post-COVID-19 syndrome: putative pathophysiology, risk factors, and treatments. Infect Dis 53: 737- 754. [Ref.]
- Halpin S, O’Connor R, Sivan M (2021) Long COVID and chronic COVID syndromes. J Med Virol 93: 1242-1243. [Ref.]
- Graham EL, Clark JR, Orban ZS, Lim PH, Szymanski AL, et al. (2021) Persistent neurologic symptoms and cognitive dysfunction in nonhospitalized Covid-19 “long haulers”. Ann Clin Transl Neurol 8: 1073- 1085. [Ref.]
- Asadi-Pooya AA, Akbari A, Emami A, Lotfi M, Rostamihosseinkhani M, et al. (2021) Long COVID syndrome-associated brain fog. J Med Virol. [Ref.]
- Stefano GB, Ptacek R, Ptackova H, Martin A, Kream RM (2021) Selective Neuronal Mitochondrial Targeting in SARS-CoV-2 Infection Affects Cognitive Processes to Induce ‘Brain Fog’ and Results in Behavioral Changes that Favor Viral Survival. Med Sci Monit 27: e930886. [Ref.]
- Wijeratne T, Crewther SJ (2020) Post-COVID 19 Neurological Syndrome (PCNS); a novel syndrome with challenges for the global neurology community. Neurol Sci 419: 117179. [Ref.]
- Camargo-Martinez W, Lozada-Martinez I, Escobar-Collazos A, Navarro-Coronado A, Moscote-Salazar L, et al. (2021) Post-COVID 19 neurological syndrome: implications for sequelae’s treatment. J Clin Neurosci 88: 219-225. [Ref.]
- Wang F, Kream RM, Stefano GB (2020) Long-term respiratory and neurological sequelae of COVID-19. Med Sci Monit 26: e928996. [Ref.]
- Soriano V, Ganado-Pinilla P, Sanchez-Santos M, Barreiro P (2020) Unveiling long COVID-19 disease. AIDS Rev 22: 227-228. [Ref.]
- Ursini F, Ciaffi J, Mancarella L, Lisi L, Brusi V, et al. (2021) Fibromyalgia: a new facet of the post-COVID-19 syndrome spectrum? Results from a web-based survey. RMD Open 7: e001735. [Ref.]
- Kaseda ET, Levine AJ (2020) Post-traumatic stress disorder: A differential diagnostic consideration for COVID-19 survivors. Clin Neuropsychol 34: 1498-1514. [Ref.]
- Taylor S, Landry CA, Paluszek MM, Fergus TA, McKay D, et al. (2020) COVID stress syndrome: concept, structure, and correlates. Depress Anxiety 37: 706-714. [Ref.]
- Lu Y, Li X, Geng D, Mei N, Wu P-Y, et al. (2020) Cerebral Micro- Structural Changes in COVID-19 Patients-An MRI-based 3-month Follow-up Study. E Clinical Medicine 25: 100484. [Ref.]
- Orrù G, Bertelloni D, Diolaiuti F, Mucci F, Di Giuseppe M, et al. (2021) Long-COVID Syndrome? A Study on the Persistence of Neurological, Psychological and Physiological Symptoms. Healthcare 9: 575. [Ref.]
- Kilinc D, van de Pasch S, Doets AY, Jacobs BC, van Vliet J, et al. (2020) Guillain-Barré syndrome after SARS-CoV-2 infection. Eur J Neurol 27: 1757-1758. [Ref.]
- Raahimi MM, Kane A, Moore CE, Alareed AW (2021) Late onset of Guillain-Barré syndrome following SARS-CoV-2 infection: part of ‘long COVID-19 syndrome?’ BMJ Case Rep 14: e240178. [Ref.]
- De Felice FG, Tovar-Moll F, Moll J, Munoz DP, Ferreira ST (2020) Severe Acute Respiratory Syndrome Coronavirus 2 (SARS-CoV-2) and the Central Nervous System. Trends Neurosci 43: 355-357. [Ref.]
- Paterson RW, Brown RL, Benjamin L, Nortley R, Wiethoff S, et al. (2020) The emerging spectrum of COVID-19 neurology: clinical, radiological and laboratory findings. Brain 143: 3104-3120. [Ref.]
Download Provisional PDF Here
Article Type: REVIEW ARTICLE
Citation: Rizzi A, Zoccolella S, Iliceto G, Centonze V, Saccia M, et al. (2022) Pathogenesis of NeuroCOVID: Views after Two Years of Pandemic. J Neurol Neurobiol 8(2): dx.doi.org/10.16966/2379-7150.185
Copyright: ©2022 Rizzi A, et al. This is an open-access article distributed under the terms of the Creative Commons Attribution License, which permits unrestricted use, distribution, and reproduction in any medium, provided the original author and source are credited.
Publication history:
SCI FORSCHEN JOURNALS
All Sci Forschen Journals are Open Access