Table 1: Table of distribution of all pair wise correlation coefficients per region by group, with p-value comparing ADHD and controls
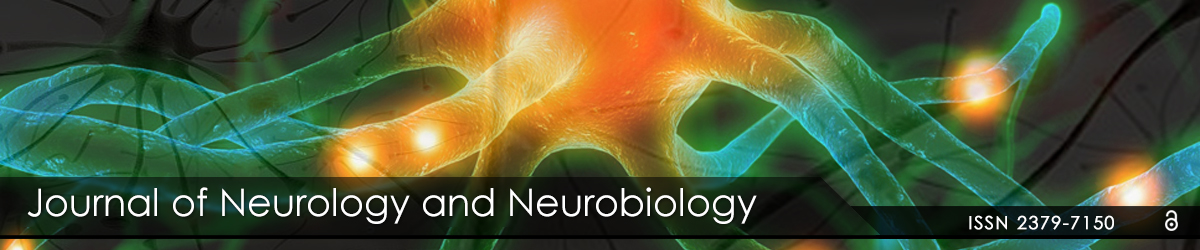
Full Text
Hilla Ben-Pazi1* Keren Rosenberg-Katz2 Lisa Deutsch3 Michal Kafri2
1Pediatric Movement Disorders, Neuropediatric Unit, Shaare Zedek Medical Center, Jerusalem, Israel2The Functional Brain Center, The Wohl Institute for Advanced Imaging, Tel Aviv Sourasky Medical Center, and Sackler Faculty of Medicine, Tel Aviv University, Tel Aviv, Israel
3Biostatistical Consulting, BioStats, Modien, Israel
*Corresponding author: Hilla Ben-Pazi, Pediatric Movement Disorders, Neuropediatric Unit, Shaare Zedek Medical Center, P.O.B. 3235, Jerusalem 91031, Israel, Tel: (972)2-6555345; Fax: (972)2-6555672; E-mail: Benpazi@gmail.com
The aim of this study was to check the correlation between brain areas in participants with attention deficit-hyperactivity- disorder (ADHD) during rhythmic motor activity. Children with ADHD have abnormal motor oscillatory activity. This maybe a result of synchronous activity and high connectivity between regions involved in motor tasks. We examined correlation between brain regions using functional-MRI in six individuals with ADHD and four controls during finger tapping (according to 1-4Hz cue). We found activation in the right cerebellum, left motor cortex, bilateral basal ganglia and left supplementary motor region. Right frontal cortex was selected as a control region. Correlation between motor regions was higher in participants with ADHD compared to controls (p=0.0046). Correlations between motor and non-motor regions were low in all participants. High correlations between motor regions but not between non- motor brain regions in participants with ADHD may reflect higher synchrony between motor regions and suggests increased, connectivity during rhythmic activity.
Motor tapping; Attention deficit-hyperactivity disorder; MRI
While clinical symptoms of “attention deficit-hyperactivity disorder (ADHD)” are well characterized and recognized the neurological underpinnings of this common neurodevelopment disorder remains unknown. Similar to neurodevelopment disorder it is thought that the cause for ADHD lies beyond localized neuroanatomical regions and is probably due to a general abnormality in brain function. Recently neuroimaging studies suggest that various disturbances in ADHD are associated with abnormal brain connectivity. Connectivity is the functional infrastructure connecting various brain regions. This system functions during activity and resting states in different ways. In children with ADHD connectivity was found to be different than age matched controls during different tasks. ADHD-related increased connectivity between cortical– subcortical areas was associated with greater impulsivity [1]. While tighter coupling was found during cognitive assignments [2], changes in connectivity during motor performance were not previously reported.
Individuals with ADHD have various motor oscillatory abnormalities that may reflect the increased connectivity during action. Children with ADHD tend to have excessive mirror overflow movements [3]. In our previous studies we found that more than half of the children with had an abnormal rhythmic response during tapping tasks; instead of tapping according to the frequency requested they tapped at a constant higher frequency [4]. This abnormal response remained constant on Methylphenidate treatment [5].
We hypothesized that individuals with ADHD will have increased synchrony between brain regions that may explain motor oscillatory abnormalities. To test this we examined individuals with ADHD and controls for correlation between brain regions during rhythmic motor tapping using functional-magnetic resonance imaging (fMRI).
Participants
Ten young adults participated in this fMRI controlled study: six with ADHD (mean 22, SD 1.3 years; 5 males) and four controls (mean 32, SD .8 years; 3 females). All participants were right handed and stated that they are able to lie still for an hour of testing. ADHD diagnosis was made by neurologists using the DSM-4 criteria. The study was approved by the Shaare Zedek Helsinki committee, and each participant signed an informed consent and was compensated for time loss and travel expenses.
Testing procedure
fMRI data was acquired using a block design paradigm. Participants tapped on an optical button immediately after the appearance of auditory stimuli with the right index finger. The auditory stimuli were presented in six frequencies (1, 2, 2.5, 3, 3.5 and 4 Hz) in blocks of 12 seconds with 6-12 seconds of rest in between. Each frequency appeared in a random order and was presented 3 times within the session. Finger tapping was tested in different frequencies in attempt to elicit an abnormal rhythmic response found in children with ADHD.
MRI data acquisition
MRI measurements were performed in a whole-body 3.0 Tesla MRI scanner (GE Signa EXCITE, Milwaukee, WI, USA). Functional protocol was based on Echo planar Gradient echo (T2* )-weighted images (GE-EPI) (TR/TE/flip angle=3000/55/90) with FOV of 24 cm2 and matrix size of 80 × 80). In addition, a 3D spoiled gradient echo (SPGR) sequence, with high resolution, was acquired for each subject to allow volume statistical analyses. Auditory stimuli were provided by shareware version of the GoldWave digital audio editor (http://www.goldwave.com) and generated by a program protocol written using Presentation 0.71 software.
Functional-MRI data analysis
MRI data was processed using Brain Voyager 4.4 software package (http://www.brainvoyager.com) [6,7]. Preprocessing steps included motion detection and correction of head motion, temporal linear trend removal and temporal high pass filter of 2 cycles per time course. 3D statistical parametric maps were calculated separately for each subject using a general linear model (GLM) [8]. Each condition (i.e. 1, 2, 2.5, 3, 3.5 and 4 Hz) was modeled separately as a boxcar regressor convolved with a canonical synthetic HRF and used in the model. Realignment parameters, reflecting head motions in six directions, were included in the model a nuisance regressors. Percent (%) signal was averaged to fit 0-1 in all regions –that did not affect correlation and Wilcoxon test. A cube was cropped (6859 pixels; 19 voxels) from each motor region of interest around the area of peak activation for regions of interest and in an area without activity for the control region. Anatomical and functional volumes were co registered and normalized to the Talairach space.
Statistical analysis
Pearson correlation coefficients were calculated between % signal values of pairs of all brain regions for the entire time course. Correlation coefficients are not expected to follow a normal distribution and several of the group comparisons have small sample sizes therefore groups were compared with a Wilcoxon two-sample test. Nominal p-values are presented.
Brain activation
All participants successfully performed the task. When comparing all tapping conditions with resting state we found activation in all participants mainly in 5 regions: Right cerebellum (Rt Cb), Left motor cortex (Lt Cx), Right basal ganglia (Rt BG), Left basal ganglia (Lt BG), Left supplementary motor region (SMA). The Right frontal cortex (Rt Frt) was selected as a control region as it was not activated during motor tasks.
Group analysis
There was no statistically significant difference between the ADHD participants and controls with respect to the level of correlation between % activation of specific brain regions pairs (Table 1).
High correlation in motor areas in ADHD participants
Participants with ADHD (Figure 1) had a higher correlation level (mean 0.46 ± 0.26) between the 5 activated motor regions (% activation in pairs of: Rt Cb, Lt Cx, Rt BG, Lt BG, SMA) compared to controls (0.35 ± 0.29; Wilcoxon two-sample test, p=0.034). Both the groups, ADHD and controls, had higher correlation levels between % activation in pairs within motor regions (mean 0.54 ± 0.21) versus motor regions and non-motor regions (mean 0.16 ± 0.20; p<0.0001). However, the correlation between % activation in pairs of motor regions was significantly higher (mean 0.60 ± 0.16) in individuals with ADHD compared to controls (mean 0.46 ± 0.26; Wilcoxon two-sample test, p=0.013). Low correlations between % activation were found in pairs of motor regions and the non-motor region and were similar in both groups (mean 0.18 ± 0.19 in ADHD versus 0.13 ± 0.22 in controls; Wilcoxon two-sample test, p=0.73; Table 1).
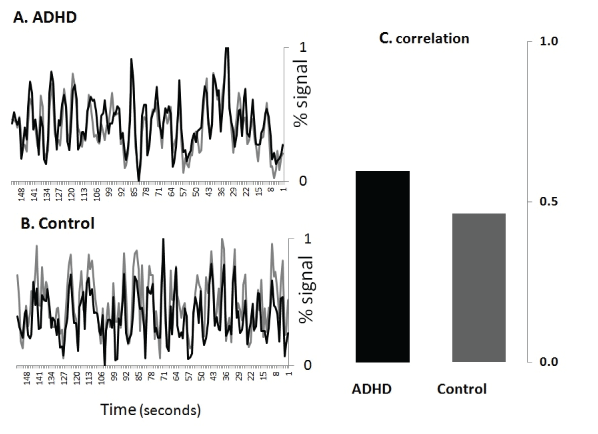
Figure 1: Correlation between motor regions during tapping task in participants with ADHD and controls. (A,B): BOLD brain activity (% signal) is presented over time (seconds) demonstrates high activation during tapping intervals and lower during rest. There is temporal correlation between the left (black) and right basal ganglia (grey) in both participants. However the participant with ADHD (A) hand higher correlation (=0.88) between left (grey) and right basal ganglia (black) than the control (B) participant (=0.54). (C): Group analysis demonstrates that participants with ADHD (n=6) have significantly higher correlations than controls (n=4) between motor regions (motor cortex, SMA, basal ganglia and cerebellum; Wilcoxon two-sample test, p=0.001).
Area analysis: high correlation within the basal ganglia
Correlation between % activation in the left and right basal ganglia was the highest (mean 0.79 ± 0.10) and correlation levels between the % activation in the right cerebellum and the right basal ganglia was the lowest (mean 0.37 ± 0.21) among the motor brain regions.
We found high correlations between motor regions but not between non- motor brain regions in participants with ADHD. The higher correlation during a rhythmic tapping detected in subjects with ADHD compared to controls reflects higher synchrony between motor regions during task engagement and may suggest increased functional connectivity during activity.
Connectivity studies in individuals with ADHD demonstrated varied results depending on task and brain region [9,10]. Resting-state fMRI studies have found connections characterized by both increased and decreased connectivity in ADHD. Decreased connectivity was found between cortical areas (e.g. posterior cingulate cortex and ventromedial prefrontal [11] and were normalized following stimulant medication [12]. Increased connectivity was found between the nucleus accumbens and the prefrontal cortex [1]. It is suggested that abnormal connectively is related with specific behavioral characteristics in ADHD. For example in ADHD children, the reward-motivation regions (striatum and anterior cingulate) had higher connectivity compared to controls [13]. Connectivity is related to the ‘default-mode network’ that may persist or intrude into periods of activity compromising attention. Increased connectivity during tasks may reflect the rest stage inadequate ability to shift from the rest stage to the attentional stage [14].
In our study the right and left basal ganglia were the area which demonstrated the highest connectivity during tapping. This increased coupling throughout motor tasks is similar to the reported coupling during non-motor assignments [2]. Thus we suggest that inability in ADHD to adjust brain connectivity levels to tasks may impact on performance. Namely while lower connectivity during rest leads to greater impulsivity [1] increased connectivity through activity may lead to lower performance of motor and non-motor tasks.
Limitations: Small sample size (n=10) and significant age difference between groups (p=0.005).
Future studies may study the role of motor training for treatment of attentional disorders. For example: biofeedback during motor activity may reflect changes in connectivity and thus enable training for gaining better control to shift from default mode to attentional mode.
This is first report, to the best of our knowledge, of increased synchrony in ADHD during a motor task. We believe that there is abnormal connectivity regulation in individuals with ADHD; maybe connectivity is abnormally low during the resting state causing inattention and increased during activities resulting in impulsivity and hyperactivity.
The authors declare that they have no conflict of interest.
This study was funded by the National Institute of Psychobiology in Israel and Shaare Zedek medical center
- Costa Dias TG, Wilson VB, Bathula DR, Iyer SP, Mills KL, et al. (2012) Reward circuit connectivity relates to delay discounting in children with attention-deficit/hyperactivity disorder. Eur Neuropsychopharmacol 23: 33-45. [Ref.]
- Massat I, Slama H, Kavec M, Linotte S, Mary A, et al. (2012) Working memory-related functional brain patterns in never medicated children with ADHD. PLoS One 7: e49392. [Ref.]
- Macneil LK, Xavier P, Garvey MA, Gilbert DL, Ranta ME, et al. (2011) Quantifying excessive mirror overflow in children with attention-deficit/ hyperactivity disorder. Neurology 76: 622-628. [Ref.]
- Ben-Pazi H, Gross-Tsur V, Bergman H, Shalev RS (2003) Abnormal rhythmic motor response in children with attention-deficit-hyperactivity disorder. Dev Med Child Neurol 45: 743-745. [Ref.]
- Ben-Pazi H, Shalev RS, Gross-Tsur V, Bergman H (2006) Age and medication effects on rhythmic responses in ADHD: possible oscillatory mechanisms? Neuropsychologia 44: 412-416. [Ref.]
- Goebel R, Khorram-Sefat D, Muckli L, Hacker H, Singer W (1998) The constructive nature of vision: direct evidence from functional magnetic resonance imaging studies of apparent motion and motion imagery. Eur J Neurosci 10: 1563-1573. [Ref.]
- Goebel R, Linden DE, Lanfermann H, Zanella FE, Singer W (1998) Functional imaging of mirror and inverse reading reveals separate coactivated networks for oculomotion and spatial transformations. Neuroreport 9: 713-719. [Ref.]
- Friston KJ, Holmes AP, Poline JB, Grasby PJ, Williams SC, et al. (1995) Analysis of fMRI time-series revisited. Neuroimage 2: 45-53. [Ref.]
- Cao Q, Shu N, An L, Wang P, Sun L, et al. (2013) Probabilistic diffusion tractography and graph theory analysis reveal abnormal white matter structural connectivity networks in drug-naive boys with attention deficit/hyperactivity disorder. J Neurosci 33: 10676-10687. [Ref.]
- Konrad A, Dielentheis TF, El Masri D, Bayerl M, Fehr C, et al. (2010) Disturbed structural connectivity is related to inattention and impulsivity in adult attention deficit hyperactivity disorder. Eur J Neurosci 31: 912-919. [Ref.]
- Fair DA, Posner J, Nagel BJ, Bathula D, Dias TG, et al. (2010) Atypical default network connectivity in youth with attention-deficit/hyperactivity disorder. Biol Psychiatry 68: 1084-1091. [Ref.]
- Li An, Xiao-Hua Ca, Qing-Jiu Ca, Li Su, Li Ya, et al. (2013) Methylphenidate Normalizes Resting-State Brain Dysfunction in Boys with Attention Deficit Hyperactivity Disorder. Neuropsychopharmacology 38: 1287-1295. [Ref.]
- Tomasi D, Volkow ND (2012) Abnormal functional connectivity in children with attention-deficit/hyperactivity disorder. Biol Psychiatry 71: 443-450. [Ref.]
- Sonuga-Barke EJ, Castellanos FX (2007) Spontaneous attentional fluctuations in impaired states and pathological conditions: a neurobiological hypothesis. Neurosci Biobehav Rev 31: 977-986. [Ref.]
Download Provisional PDF Here
Article Type: Research Article
Citation: Ben-Pazi H, Rosenberg-Katz K, Deutsch L, Kafri M (2016) High Correlation during Motor Tapping in Young Adults with Attention DeficitHyperactivity Disorder: A Controlled Functional MRI Study. J Neurol Neurobiol 2(4): doi http://dx.doi. org/10.16966/2379-7150.129
Copyright: © 2016 Ben-Pazi H, et al. This is an open-access article distributed under the terms of the Creative Commons Attribution License, which permits unrestricted use, distribution, and reproduction in any medium, provided the original author and source are credited.
Publication history:
SCI FORSCHEN JOURNALS
All Sci Forschen Journals are Open Access