Figure 1: AI toolbox for understanding bio‐physicochemical identity at the nano-bio interface. The model envisions complementing and predicting the core properties of nanomaterials to identify the nano-bio interaction sides. After the selection of appropriate NP properties, real experimental data must be used for ML model development, optimization, and cross‐validation before unknown NP properties or the nano-bio interaction can be predicted. The boundary condition indicated by radial outlines (right panel) represents working spaces to apply AI and ML approaches to predict unknown properties for the prediction of toxicity and health effects in nanomedicine.
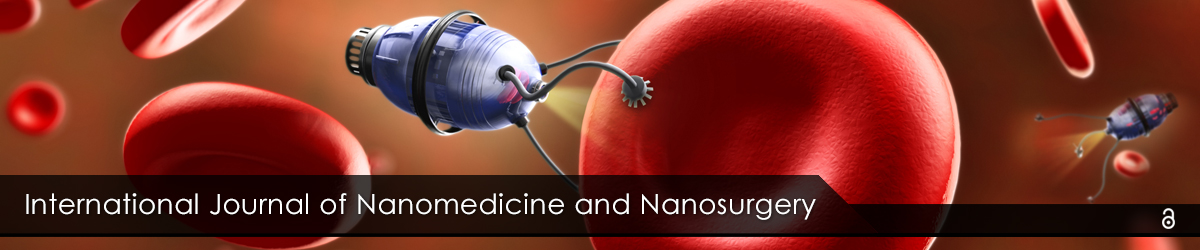
Full Text
Prof. Dr. Seyed Saeid Zamanieh Shahri, MD* Prof. Dr. Sonia Sayyedalhosseini, MD*
Faculty Members in California Northstate University, CNSU-University Professors in Losrios Community College District, USA*Corresponding author: Prof. Dr. Seyed Saeid Zamanieh Shahri, MD, Faculty Member in California Northstate University, CNSU-University Professor in Losrios Community College District, USA, Tel: (916) 724-9485; E-mail: saeid.zamanieh@cnsu.edu; zamanis@flc.losrios.edu
Prof. Dr. Sonia Sayyedalhosseini, MD, Faculty Member in California Northstate University, CNSU-University Professor in Losrios Community College District, USA, Tel: (916) 517-8294; E-mail: sonia.sayyedalhosseini@cnsu.edu; sayyeds@flc.losrios.edu
Today, in accordance with the new pandemic conditions of the coronavirus and the need to do most of the work online and virtually, telemedicine is recognized as an effective way to provide quality health care services without time and space constraints. This review article could be a step toward more awareness and application of this technology in today’s medicine. Generally based on the positive experiences of patients and clinicians from the enforced telemedicine-based care during the COVID-19 pandemic, unique insight emerged. Integration of telemedicine-based care into daily practice may have particular importance for patients with rare cancers and those who live distant from care centers. Enforced adoption of telemedicine has demonstrated the time- and cost-saving implications for patients, which have the potential to revolutionize cancer care delivery [1]. Researches show that future developments in the field of telemedicine are seriously influenced by technological advances in the three areas of mobile communications, vital sensors and nanotechnology. Nano and micro robots are various helpful medical devices in science and health knowledge [2]. Nanorobotics is also a new field of science that includes the design, construction and programming of nano robots. The scope of nano robots within the human body is very wide. Nano robots are able to examine a specific tissue and study its biochemical and biomechanical properties in great details, and in general, they can easily identify the biological environment. So, comprehensive view of the capabilities and capacities of telemedicine and nanoparticles in medicine to apply these technologies in providing remote health services could be helpful.
Telemedicine; Nanotechnology; Nano robot; Micro robot
COVID-19: Corona Virus Disease 2019; RMH: Royal Marsden Hospital; ED: Emergency Department; C. reinhardtii: Chlamydomonas reinhardtii; E. coli: Escherichia coli; 3D: 3 Dimensional; SPIONs: Super Paramagnetic Iron Oxide Nanoparticles; MRI: Magnetic Resonance Imaging; PET: Positron Emission Tomography; NIRF: Near-Infra Red Fluorescence; MSCs: Mesenchymal Stem Cells; AIDS: Acquired Immuno Deficiency Syndrome; EPR: Enhanced Permeability and Retention; SiRNA: Small interfering RNA; CTC: Circulating Tumor Cell; GMPs: Gold Micro Plates; BSA: Bovine Serum Albumin; PCL: Paclitaxel; MCF-7: Michigan Cancer Foundation-7; NPs: Nano Particles; ToF-SIMS: Time-of-Flight Secondary Ion Mass Spectrometry; Au: gold; TiO2: Titanium di Oxide; NMs: Nano Materials; Van@GO: Vancomycin conjugated with Graphene Oxide; VRSA: Vancomycin-Resistant Staphylococcus aureus; AFM: Atomic Force Microscopy; STM: Scanning Tunneling Microscopy; DNA: Deoxyribo Nucleic Acid; AI: Artificial Intelligence; ML: Machine Learning; MBioFs: Metal-Biomolecule Frameworks; i-TASSER: iterative Threading Assembly Refinement; CSI: Cell Shape Index; NAFs: Nuclear Area Factors
Telemedicine or telehealth is the use of medical information to improve patient’s health. Reorganization in healthcare delivery, financing, and advancement in electronic health records and clinical decision support systems can accelerate the telehealth adoption into healthcare delivery. Robotic technologies can play an important role in the use and delivery of telemedicine during coronavirus disease (COVID-19) and the post-pandemic world [3].
One of the applications of micro robots in medicine is the “Micro robot capsule” in endoscopic operations that receives images from the gastrointestinal tract. The images received from it can not only be examined in the presence of the patient, but also in combination with telemedicine systems, the images can be sent to a specialist doctor anywhere in the world and based on it, diagnosis, instructions and even medication can be received remotely.
In addition, the use of nano robots or bio nano robots in drug delivery reduces the side effects of drugs and speeds up the healing process. Surgeon nano robots can also travel to areas of the body that humans and ordinary instruments cannot reach. Work has also been done on nano robots that increase the immune system against diseases, and some nano robots can also be used to identify an area of chemical contamination in the body.
However, what is certain is that according to Nono capabilities, many nano robots and micro robots can be used well in the form of telemedicine systems. In addition to the applications of these technologies in providing remote health services at the community level, the services of these advanced technologies, can be used especially in the field of human remote monitoring and treatment for isolated and inaccessible places such as quarantine, special military environments and hard-to-reach places as alternative methods to provide specialized health services.
By comprehensive view of the capabilities and capacities of telemedicine and micronutrients in medicine, we can reach a new approach to integrating the potential capacities of these two parts of advanced technologies to achieve new applications to find one of these technologies in providing health services, especially remote health services.
In the study by Smrke A, et al. of 379 planned face-to-face appointments, 283 (75%) were converted to telemedicine. Face-toface appointments remained for patients who needed urgent start of therapy or performance status assessment. Patients lived on average >1.5 hours from Royal Marsden Hospital (RMH). Patient satisfaction (n=108) with telemedicine was high (mean, 9/10), and only 48% (n=52/108) would not want to hear bad news using telemedicine. Clinicians found telemedicine efficient, with no associated increased workload, compared with face-to-face appointments [1].
Nanotechnology in medical engineering has revolutionized the treatment of diseases such as cancer, cardiovascular disease, neurological diseases, infections and other diseases. Nanotechnology has created small, fast and inexpensive tools with new functions. Various evolutions in science by nanotechnology, sensors and computers have led to the emergence of using nano and micro robots. On one hand nano robots, deal with large volumes of information and, on the other hand, communicate with the physical world through sensors and operators [4].
By using nano models and biomolecular calculations, it seems that nano robots will be able to do pre-programmed tasks correctly. Therefore, it can be hoped that in the coming years, nano robots will be used for remote surgery or will constantly monitor the human body and whenever an organ is damaged; it will be immediately identified and repaired [5].
To demonstrate the capabilities of this system, the researchers performed various experiments on a case in which nano robots used different methods to identify proteins in vessels of varying dimensions and sizes. Finally, as expected, the results showed that nano robots were better able to detect a specific target in smaller vessels. In addition, the use of heat and chemical sensors had a great impact on the qualitative and quantitative development of nano robots. Many researchers are now equipping their robots with wireless capability, which has several advantages over single robots [5].
As Hollander JE, et al. [6] mentioned in the research in Telemedicine for COVID-19 “No telemedicine program can be created overnight, but U.S. health systems that have already implemented telemedical innovations can leverage them for the response to COVID-19. A central strategy for health care surge control is “forward triage”-the sorting of patients before they arrive in the Emergency Department (ED). Direct-to-consumer (or on-demand) telemedicine, a 21stcentury approach to forward triage that allows patients to be efficiently screened, is both patient-centered and conducive to self-quarantine, and it protects patients, clinicians, and the community from exposure” [6].
An important goal for remote clinical triage centers is the ability to provide case clarity on the first call, so that a phone call is managed without the use of other services, or that the caller only communicates with a call transfer to the person concerned. The called resolution indicates that the initial call responds well to the caller by adapting care to the medical need.
Mobile health clinics introduce cost-effective and usable conditions with the possibility of transmitting clinical information to the physician remotely so that the physician can make an accurate decision [7].
A good remote patient monitoring system would also help to report on Medicare’s transitional care management initiative which is another revenue producing avenue. Telemedicine, together with remote patient monitoring, help providers keep their patients engaged and connected while producing two separate revenue streams at a time when in-office visits may be down [8].
This algorithm consists of five torque modules, that the homogeneous matrix, robot and the common parameters are well defined. Dynamic motion equations have been performed by using Lagrange methods to simulate numerical calculation for the required torques for the motion of each joint according to the defined path [9].
Micro robots are controlled by a magnetic field and can move in living tissues and organs through the body’s bloodstream. They have also made many potential applications in the field of medical engineering. For example, they may be performed for surgery on blood vessels, which is expected to be one of the medical methods adopted in the near future. Micro robots may be used in many small spaces to eliminate the need to open and close the surgical site [4,9]. Nanorobotics is a new science that involves the design, fabrication, and programming of nano robots that could be about 1.5 nm and it is able to count specific molecules in a chemical sample used in medical technologies. The scope of work of nano robots is inside the human body and they can display the amount of different compounds in the body and store information in their internal memory. Nano robots are able to examine a specific tissue and study the biochemical and biomechanical properties in great detail, and in general, easily identify the biological environment [4].
The potential medical applications of nano robots range from eliminating the side effects of chemotherapy to treatment of Alzheimer’s disease. Various researchers have made great strides in developing the systems needed to develop active and useful nano robots such as sensors, data transmission systems, and fuel systems. But much remains to be done to study the idea of molecular systems that can travel through the body’s arteries to identify and treat disease [5].
The driving force for movement and travel throughout the body is through the bloodstream. Another important factor is the safety of the patient. External navigation systems are used to control nano robots and transfer information through different software in telemedicine. So, the information can be transferred even to other parts of the world [4,5]. Nano robots can be identified and directed to the destination by using ultrasonic signals which are transmitted to the body and reflected back to the original source. Ultrasound signals travel to the patient’s body and the pulse is guided by ultrasonic signals, and doctors determine its path, which can be done remotely wirelessly and can also be used in telemedicine [10].
With the help of cell repair nano machines, problems related to cells and tissues can be solved. For this purpose, cell repair machines need sensory devices and receptors in molecular dimensions. The size of these machines is equal to the size of bacteria and viruses. Cell repair machines can move in the path of blood flow and just like viruses that can enter body cells they enter the cells. Nano machines identify existing problems by testing the content and activity of cells [11].
Using the special properties of biological organisms and various living biological materials has been used to perform the desired functions for providing nano sized biostructures.
Recent developments in biological cell-driven biohybrid microswimmers revealed that different type biological microswimmers (e.g., bacteria and algae) populations need to interact fluidically with each other in the same fluidic media. Base on this fact the study on the swimming behavior of two opposite types of biological populations: Chlamydomonas reinhardtii (C. reinhardtii) algae (puller-type microswimmers) population in coculture with Escherichia coli (E. coli) bacteria (pusher-type microswimmers) population, discovered that the shift in motility behaviors of these two opposite-type swimmers suspended in the same fluidic media [12].
Singh AV, et al. proposed a facile surface patterning technique and a specific, strong biotin-streptavidin bonding of bacteria on patterned surfaces to fabricate Janus particles that are propelled by the attached bacteria. Such bacteria-driven Janus microswimmers could be used for future medicine in targeted drug delivery and environmental remediation [13].
Spermbots as another new class of microrobots that created by coupling sperm cells to mechanical loads, utilize the flagellar movement for propulsion and do not require any toxic fuel in their environment. They are also naturally biocompatible and show considerable speed of motion thereby giving us an option to overcome the two challenges of propulsion and biocompatibility. Some other challenges in this regards are unequal force of motion in all sperm cells, the load couldn’t be toxic, and load attachment is low-yielding and random and can be attacked by microbes. In conclusion, spermbots show very promising traits that could open up exciting new applications in the medical field, especially in the female reproductive tract. The aim of spermbot research community should be to develop spermbot which could operate autonomously in swarms, while still giving the surgeons an option to control them [14].
Wirelessly controlled, mobile, untethered milli/microrobots present a promising future for medical interventions and biomedical engineering, and increasing the quality of life by reducing pain, recovery time, and cost. Modeling and design of microrobots depends upon the operational environments and tasks they are intended to perform. Such designs need collaborative effort between clinicians and robotic researchers, chemists, biomedical engineers, and materials scientists. Materials and fabrication need expertise to understand where biocompatibility, mechanical resilience and durability are all important in the dynamic in vivo physiological environments. Bioinspired approaches could provide critical solutions to physicochemical challenges. For example microrobot mimicking erythrocytes, which could exhibit shape memory and adaptability under applied stress in large vessels, and load bearing tissues without undergoing plastic deformation, had more adaptability for systemic circulation. In vivo theranostic functionality needs 3D navigation with on-board power consumption, mimicking dynamic organ motion such as respiration, heart beating, and peristalsis. 3D fabrication technologies enabling milli/microrobot fabrication at the single cell scale are empowering high resolution visual imaging and in vivo manipulation capabilities. Many invasive excision and incision based diagnostic biopsy, prostrate, and nephrolgical procedures can be performed minimally or almost noninvasively due to recent advancements in microrobotic technology [15].
Further wireless cross communication between groups of microrobots or transceivers inside the human body and the clinician to assess safe use is the next big challenge. Nonetheless, due to growth in research and funding, now is an appropriate time to think critically and creatively about the potential pitfalls and opportunities inherent in microrobotic theranostic applications in pharmaceutical design [15].
The cancer nanomedicine field has expanded exponentially in recent years. In stark contrast to the numerous new materials and papers that are being produced, only about a dozen nanomedicinal anticancer drugs (antibody-drug conjugates included) have thus far made it to the market. To change this situation, we must move away from continuously making increasingly complex nanomedicine materials and critically reconsider how we are doing translational cancer nanomedicine research [16].
Nano machines determine whether a cell needs to be repaired or destroyed, depending on the problem. This method is also used to treat cancer. In addition, the control of these nano machines is done by nano computers [5].
One of the things that cannot be treated by nanotechnology is related to mental health. However, some mental abilities are treated by restoring the brain’s hormonal and chemical levels. The problem of aging is also solved by nano machines. If cell repair machines can repair damaged cells and structures, the aging process will be much smoother [6].
Capsule energy supply and location of the capsule in the body
One of the applications of micro robots in medicine is the “Micro robot capsule” in endoscopic operations that receives images from the gastrointestinal tract. The endoscopic capsule works using 2 batteries that can be generated for 8 hours to capture images at 2 frames per second, and the number of frames is expected to improve as well. In order to determine the position of the oral capsule inside the gastrointestinal tract as a function, the physician must know whether the information collected is correct or not? [17,18].
To determine the location of the capsule, an array of sensors are connected at a suitable distance from each other, which is to estimate the distance between the capsule and its location. However, in fact this method is quite vague, because different tissues in the body have different dielectric constants, which leads to differences in signal attenuation. Another problem is that sometimes the capsule position information system may be lost [18].
Using a magnetic field to optimize the detection range
A permanent magnet is attached to the capsule to detect the strength of the magnetic field. Magnetic field strength sensors are 1 cm accurate and 11 cm deep. Therefore, the detection range is optimized [17]. There are several advantages in magnetic field detection; this system has a permanent magnet, there is also a full-time magnetic field that determines whether the signal information is transmitted or not?
In the study Singh AV, et al. proposed hairbots as microrobot biogenic hair, as a unique multifunctional material for identifying compounds of natural origin for microrobotics. Further, in his study, when hairbot loaded with Super paramagnetic Iron Oxide Nanoparticles (SPIONs), it became responsive to magnetic fields and gradients. Ajay used Ironoxide nanoparticles because of their potential to be used in diagnostics as probes in Magnetic Resonance Imaging (MRI), Positron Emission Tomography (PET) and Near-Infrared Fluorescence (NIRF) imaging. Another unique characteristic of hairbots was having a central medullary hollow space that made it also to be as Ultrasound contrast agents. Along with the drug carrying capability of hairbots, SPION coated hairbots are well suited for targeted and minimally invasive therapies [19].
External magnetic fields were used to enhance differentiation of Mesenchymal Stem Cells (MSCs) into bone like cells, which were used as magnetic therapy for bone healing. The action of hairbots as osteoconductive material triggered osteogenic differentiations of MSCs. And also, due to its hollow medullary region, hairbots were designed to perform theranostic dual functions (therapy + diagnostic)-as Doxorubicin drug delivery vehicle, and for ultrasound contrast imaging, that provided a novel material in the search of new multifunctional microrobots [19].
Using nano robots for surgery
Controllable nano robots that can be used in cancer surgery are developed in Britannia. The high precision of this nano robot is just one of the benefits. Inside the device are very small motors that enable the device to travel to a specific point in the tumor. However, with the same surgical device, it is not possible to work on tumors with millimeter accuracy. But nano robots increase this precision to the nano. With this nano robot, pathology tests or medical diagnosis can be performed in a few minutes, and even a drug can be inserted into the patient’s body in order to kill the tumor accurately. The use of nano robots does not mean that surgeons are excluded from the surgical process, but that surgeons should monitor the activity of nano robots during surgery and act as a pilot in this direction [20].
Making carbon nano robots to carry drugs in patients’ bodies
Carbon nano robots measuring 100 nanometers guide injected drugs into patients’ tissues by means of controlled devices. Carbon nano robots can carry any drug in the body. After injecting the drug into this device, the drug reaches all the diseased tissues, especially cancerous and AIDS tissues, and destroys them. At present, computer simulation of this design has been performed [20].
Nanotechnology offers several attractive design features that have prompted its exploration for cancer diagnosis and treatment. Role of Enhanced Permeability And Retention (EPR) in cancer barrier is somewhat oversell considering less than 1% nanomedicine formulations follow the trend designed in lieu of EPR. In the last decades, it has been increasingly recognized that there is large interand intra-individual heterogeneity in EPR-mediated tumor targeting, explaining the heterogeneous outcomes of clinical trials in which nanomedicine formulations have been evaluated. The EPR effect is the mechanism by which high-molecular weight non-targeted drugs and prodrugs accumulate in tissues that offer increased vascular permeability, such as in sites of inflammation or cancer. The EPR effect is the phenomenon used by nanocarriers to deal with cancer tissues. To support cancerous growth, neovascularization occurs constantly.
So, the EPR effect of nanoparticles has become the gold standard principle for cancer drug delivery systems, as safe, simple and effective therapy. By this means, efforts should be provided to overcome these barriers allowing the enhancement of nanomedicines on the market with the following strategies:
(i) The normalization of the tumor vasculature;
(ii) The possibility to include collagenase or hyaluronidase into the tumor microenvironment to simultaneously improve the transport and reduce the interstitial fluid pressure and
(iii) The increase of the tumor permeability using external or internal stimuli [21].
In another study Singh AV, et al. used E. coli microswimmer that combines the active locomotion and sensing capabilities of bacteria with the desirable encapsulation and viscoelastic properties of a soft double-micelle microemulsion for active transport and delivery of cargo (e.g., imaging agents, genes, and drugs) to living cells. They used Quasi-monodisperse double emulsions with an aqueous core that encapsulated the fluorescence imaging agents, as a cargo and streptavidin oil shell for attachment of biotin-conjugated E. coli. Their study as an in vitro model demonstrated the feasibility of the soft microswimmers that offered it as applications in delivery of imaging agents, drugs, stem cells, siRNA, and therapeutic genes to live tissue in in vitro disease models and stagnant or low-flow-velocity fluidic regions of the human body [22].
The use of liquid and tissue biopsy biomarkers is less straightforward in the case of passively targeted nanomedicines, as there are no surface receptors available for immunohistochemical staining or Circulating Tumor Cell (CTC) assessment. Accordingly, these nanomedicines likely require imaging probes and protocols for patient stratification. Several recent studies have established companion nanodiagnostics and nanotheranostics to address EPR effect heterogeneity and to predict nanotherapy outcomes [16].
Investigation of the behavior of nano robots in the environment
The blood contains many cells that each cell is about a few microns in diameter. One of the characteristics of nano robots is the movement in the blood. The physical behavior of nano robots is completely different from the performance of large robots [6].
The fluid velocity in blood capillaries (20 µm in diameter) is 1 mm/ sec [23], which seems to be reasonable for the movement of a nano robot. Therefore, the use of higher speed depends on the intended purpose and application of nano robots. In the case of using a set of nano robots, the communication between each one of them is done by chemical connections. Generating a signal with a small amplitude and low noise commensurate with the biological conditions of the cell can destroy the target cell or destructive cells, which requires bioprocessing and a high level of intelligence in a nano robot [20].
Nano robot should have a sufficiently desirable design with its performance in a liquid environment with a high degree of freedom. The nano robots proposed for the design include biological models with the components and molecular rotation pattern. In general, a robot arm has been used to perform special operations, but in this regard, all biological conditions such as protein uptake and care of the system against attack, as well as the compatibility with the environment, should be examined [20].
The control bell of the systems will be based on the performance of sensors embedded on the nano robot. A set of sensors should monitor the working environment and analyze the possibility of operation in capillaries or arteries. Making the best sensors and activating them in nanoscale is a complex matter that requires the help of chemical sensors [11]. During the 2010s, three main designs of nano robots have emerged: helices (also called nanoswimmers), nanorods (also called nanoswimmers, Nano motors or, if longer, nanowires) and DNA nano robots [20].
Recently researchers designed different type of nano/micro robots, that in this review article some of them are mentioned.
Gold microplates
Nowadays according to Singh AV, et al. studies a facile biomineralization route for Gold Microplates (GMPs) synthesis used Bovine Serum Albumin (BSA) as a reductant. So, by adding BSA to HAuCl4 solution yielded spontaneous versatile anisotropic and partially hollow GMPs upon aging. In their study the instantaneous protein denaturation at low pH enabled access to serine and threonine hydroxyl, and sulfhydryl groups of BSA, acts as a reductant and stabilizer, respectively. Transmission electron microscopy/Xray diffraction studies revealed highly crystalline and anisotropic structures (triangle, pentagon, and rectangle). Atomic force microscopy/scanning electron microscopy analyses demonstrated unique morphology of microplates with a partially void core and BSA mineralized edge structure. Macrophage-microplate interaction studies using live cell confocal imaging revealed that cells are capable of selectively internalizing smaller GMPs. Large GMPs are preferentially picked with sharp vertices but cannot be internalized and exhibit frustrated phagocytosis-like phenomenon. They explored particle phagocytosis as an actin mediated process that recruits phagosome-like acidic organelles, shown by a lysosensor probe technique. The biocompatible GMPs exhibited ~70% paclitaxel (PCL) loading and sustained release of PCL, showing antitumor activity with the Michigan Cancer Foundation-7 (MCF-7) cell line, and could be a novel drug carrier for breast cancer therapy [24].
Gold nanoparticles
So far, little is known about how the surrounding chemical environment influences the extracellular biomineralization at the cell membrane interface and what effect this then has on uptake and biocompatibility. Recently, a novel technique to synthesize versatile 0, 1, and 2 dimensional gold nanostructures under ambient synthesis conditions was published. For example, cancer cells are known to synthesize Nano Particles (NPs) de novo from Au ions. The biomolecular imaging of cell NP interactions using time-offlight secondary ion mass spectrometry (ToF-SIMS) represented an evolving tool in nanotoxicology. Singh AV, et al. in Germany applied ToF-SIMS 3D imaging to map the intracellular and protein-mediated biomineralization of gold (Au) ions into spherical and anisotropic Au nanoparticles (Au-NPs) in A549 cancer cells under controlled [25].
Their novel approach was used to model the resistance of human alveolar A549 cells against gold (Au) ion toxicity through intra- and extracellular biomineralization. Their findings demonstrated the potential of ToF-SIMS 3D imaging to better understand cell-NP interactions and their impact in nanotoxicology. It also signified a novel tool to probe the cellular biochemical microenvironment and could be extended further to elucidate the toxic effects of particles in the cell’s neighborhood [25].
Magnetic nanomaterials
High aspect ratio magnetic nanomaterials possess anisotropic properties that make them attractive for biological applications. Their elongated shape enables multivalent interactions with receptors through the introduction of multiple targeting units on their surface, thus enhancing cell internalization. Moreover, due to their magnetic anisotropy, high aspect ratio nanomaterials can outperform their spherical analogues as contrast agents for MRI applications.
The two main synthetic routes for the preparation of anisotropic magnetic nanomaterials are:
1) Direct synthesis (in which the anisotropic growth is directed by tuning the reaction conditions or by using templates).
2) Assembly methods (in which the high aspect ratio is achieved by assembly from individual building blocks).
That provides the biomedical applications of anisotropic magnetic nanomaterials for magnetic separation, detection, targeted delivery and magnetic resonance imaging [26].
Metallic nanoparticles
In recent years, biogenic synthesis process of metallic nanoparticle has attracted considerable attention. In biogenic synthesis process, the synthesis of nanoparticles is achieved through microorganisms and plants. The biosynthesis may actually provide nanoparticles of a better defined size and morphology as compared to some of other physicochemical methods of production. It has been found that the microbial-based synthesis process is readily scalable, eco-friendly, and compatible with the use of product for pharmacological applications, but production through microorganisms is often more expensive than the production of plant-based materials [27].
Titanium dioxide nanomaterial
Another study by Meyer T, at al. was done related to adverse effect, simultaneous quantification and visualization of Titanium dioxide (TiO2) uptake at the single cell level in an in vitro model of the human small intestine. TiO2 is used as food additives. Consequently, humans could be exposed to high amounts of Nanomaterials (NMs) that may induce adverse effects of chronic exposure conditions. Advanced quantitative imaging tools for NM detection within biological environments are required. A combination of the label‐free spatially resolved dosimetric tools, microresolved particle induced X‐ray emission and Rutherford backscattering, together with high resolution imaging techniques are applied to visualize the cellular translocation pattern of TiO2 NMs and to quantify the NM‐load in the single cell level [28].
Gold microplate-macrophage
Biological systems have intriguing capabilities to synthesize and assemble a range of inorganic ions into intricate biomineral anisotropic structures such as calcite, amorphous silica (diatoms), copper (E. coli) and magnetite (magneto-tactic bacteria). The sophisticated natural biominerals are not only on a micro- to nanoscale but also few in number (e.g.,Si/Ca), which often limits their technological expansion in to biomedicine applications of biomineralized structures. Essentially, these limitations are due to a lack in diversity in the availability of inorganic minerals, shapes, and synthesis routes. As a proof-ofconcept of study by Singh AV, et al., [25] their protocol demonstrated the feasibility of biocompatible BSA-gold microplate production with internal volume, which puts forth an interesting perspective as drug delivery carriers.
The limitation of this method is probability to block the smaller blood vessels when used for systemic delivery because of its polyhedron shape with sharp tips and micrometer size. In this context, protein is an excellent natural building block and has attracted much interest as a stabilizing agent to prepare microparticles due to its versatile functionality and excellent biocompatibility [24].
Polymeric-based nanoparticles
Biofilms are highly tolerant to antimicrobial agents and adverse environmental conditions being important reservoirs for chronic and hard-to-treat infections. Nanomaterials exhibit microbiostatic/ microbicidal/antipathogenic properties and can be also used for the delivery of antibiofilm agents.
Nanoparticles based on biodegradable and biocompatible polymers, in particular, have recently shown potential in cancer therapy and as sustained drug-delivery vehicles, prolonging drug half-life, improving solubility, and reducing immunogenicity. Furthermore, simultaneous co-delivery of multiple drugs, enhancing synergistic effects of certain drugs and suppressing drug resistance and wound-healing applications via delivery of bioactive agents, become possible with these nanoparticles [29].
Vancomycin conjugated with graphene oxide (Van@GO)
In the other study by Singh V, et al. regarding “Graphene Oxide Synergistically Enhances Antibiotic Efficacy in Vancomycin-Resistant Staphylococcus aureus” the results clearly exhibited the enhanced inhibition activity of Van@GO compared to Vancomycin alone against Vancomycin-Resistant Staphylococcus aureus (VRSA). The high surface area of graphene oxide facilitates high loading and multivalent interaction of conjugated Vancomycin leading to polyvalent inhibition. Further, they found that Van@GO significantly reduces the motility of VRSA via inducing oxidative stress compared with untreated samples. Their findings highlighted the importance of Van@GO as an effective polyvalent inhibition recipe for VRSA [30].
Control and intelligence can be implemented in two ways. But figuring out which one is really more practical needs more in-depth study [11].
A method that is referred to as molecular application and the use of motors and sensors in molecular dimensions, is based on the fact that the sensors, by receiving and sending chemical changes, control the entry and exit of information in a nano robot and also from nano electric techniques are used to process this information outside the body or inside nano robots and inside the body’s arteries [11].
The second solution is to use the biotechnology technique that is currently being used to produce and modify products in plants and animals and the production of microorganisms for special applications. By using DNA, an intelligent structure can be created for a nano robot [11].
By encrypting the digital information inside the DNA, after receiving the information, the nano robot can process the information in itself and send the necessary commands. Due to the size of the DNA fragments, a base pair of DNA that is equivalent to 0.33 nm, can store a large amount of information in a very small space. In the second method, new biological conditions can even be defined by a nano robot for a cell [6].
DNA molecules as fundamental building block bring about various stimuli-responsive mechanisms and elementary functions that can be integrated into solving sophisticated tasks in a preprogrammed manner. In other words, DNA nanotechnology is encroaching on the realm of robotics [31].
Advantages of DNA over other smart materials in the construction of robots are the intrinsically biocompatibility and biodegradability, which lightens the concerns on its possible adverse effects. On top of this, the marvelous development in automated DNA synthesis has enabled production of DNA strands in large quantities, yet with sitespecific addressability, which may lead to integrated functionality, such as drug-loading, site-targeting and stimuli-responsiveness within one rationally designed architecture [32].
Although electrostatic interactions are important for all biological macromolecules, their energetic consequences are enormous for nucleic acids. Consider that only 5 of the 20 amino acids that make up proteins are charged at physiological pH, and as there are both positively and negatively charged amino acids, the net charge on most proteins tends to be small. In contrast, the phosphate backbone of nucleic acids carries one negative charge per residue. As a result, nucleic acids are highly charged. Bringing these charges in close proximity during RNA folding or DNA compaction requires overcoming an enormous electrostatic energy barrier [33].
Water molecules surround the DNA in a very specific way that, on the one hand, the texture of this hydration shell depends on the water content and, on the other hand, actually influences the structure of the genetic substance itself. These findings are not only important in understanding the biological function of DNA; they could also be used for the construction of new DNA-based materials [34].
From the point of view of the Fayman project; nano robots in scale 1 to 100 nanometers, can produce other machines, from the bottom up by arranging atoms next to each other which is well known as assembler and this production process named self-assembly. An assembler can carry all the instructions and information needed to make a replica, in which case it is called a replicator or nano-replicator. Nanotechnology oversees the creation and control of these assemblers and replicators. These assemblers and replicators themselves are able to embed a multitude of nano robots and, through this massification, create an activity in the molecular scale [35].
This understanding has been inspired by nanotechnology in natural biological devices. Nanotechnology creates devices, materials and manipulating their order and performance from the bottom up, means in the atomic and molecular scale. From this perspective, the nanoscale is not just a super-scale or a step in downsizing, but it is the most basic scale in which matter in its qualitative forms is designed and the rules governing its operation are settled. This will complement the current nanorobotics manufacturing via top-down approaches used for targeting the therapeutics to inaccessible sites in vivo. It will be essentially 3D chemical printing of a large functional molecular structure from basic biochemical building blocks, one atom at a time [35] [As nanoparticles are frequently synthesized by either top-down or bottom-up approaches. Top-down approach is based on the mechanical methods of size reduction by breaking down the bulk materials gradually to nanosized structures. Bottom–up approaches are based on the assembly of atoms or molecules to molecular structure in nanoscale range [27]].
The future clinicians might be able to develop synthetic micro/ nanorobots for the personalized medicine due to current boom into bioprinting and machine-learning tools. Programmable matter represents the idea that matter can change its shape and physical properties just by reconfiguring its atoms using cutting-edge tools such as Atomic Force Microscopy (AFM) and Scanning Tunneling Microscopy (STM). Developments in DNA nano-origami will enable researchers to fabricate, program and control the matter at the atomic level; this would massively help in scaling up nanorobot productions [35].
For cancer researchers, the ability to access nanoscale devices into living cells allows them to gain unique knowledge for both the clinical field and the basic knowledge field. The ability to interact simultaneously, on a molecular scale, with a number of proteins and nucleic acids, provides a better understanding of the complex patterns of regulation and signaling of cell behavior under normal and abnormal states, through this channel leads to the development of cancer in molecular scale.
Nature has developed diverse mechanisms to achieve motion at small scales. Many microorganisms possess chemical rotors that enable them to power flagella or cilia, actuated to produce a corkscrew or beading motion leading into locomotion [18]. Such propulsion mechanism has been the inspiration for rotating synthetic micro robots, where synthetic helical microstructures, flexible filaments, or tumblers rotate in axis to that of a bacterial flagellum. Each individual micro robot is energetically independent of the other micro robots rather than been dragged toward the direction given by the magnetic field. Ultrasound is another external energy input to power micro robots. More recent developments have utilized high intensity focused ultrasound to induce the rapid vaporization of chemical fuels that result in bullet‐like motion micro robots and the use of a traveling wave to induce the oscillation of a bubble trapped within a micro robots structure [17].
The most effective way for nano robots to be constantly moving and active (in any environment, not just a biological one) is to generate energy sources from the environment in which the Nano robots operate: fluid kinetic energy and electromagnetic radiation which are emitted from light, are suitable options for the energy source for open work space [11].
Also, the use of temperature and light changes is suitable options. But for a large workspace, the energy produced by the vibration will be more suitable that can be used in various environments, including the biological environment [11].
In light of these developments, incorporation of key nanomedicine concepts into the general medical curriculum ought to be considered. Contemporary medicine is becoming increasingly dynamic, with the expectation that rapid advances in nanomedicine research and associated technologies are likely to profoundly impact the conduct of future medical practice. Given the pace with which such developments are occurring, the comparative lack of familiarity held by many current and prospective doctors with regard to nanomedicine suggests an increasingly urgent need for education and training in this field. While the ensuing commentary encompasses the necessity of continuing professional development activities in nanomedicine for current medical professionals, primary focus is given here to the medical education of future doctors [36].
Within the existing general medical curriculum, issues pertaining to potential ethical concerns in nanomedicine may be accommodated in a standard professional medical ethics class that utilizes, for example, Beauchamp and Childress’ widely cited four principles of biomedical ethics, i.e., respect for autonomy, beneficence, non-maleficence, and justice. Given the critical importance of developing effective skills in doctor-patient communication and the practical inseparability of communication skills and ethical behaviors, it also is likely that potential ethical concerns in nanomedicine could be addressed in a number of professional communication and clinical skills courses offered in various general medical curricula. Since, nanomedicine distinctly is beginning to influence the way in which we think about future medical therapies and professional practice, it now becomes incumbent on medical educators to begin giving consideration to the manner in which relevant principles and concepts might be incorporated into the general medical curriculum [37].
The correlation of in vitro generated pharmacokinetics and pharmacodynamics to in vivo application scenarios is an important step toward the development of safe nanomedicinal products. So Singh AV, et al. worked on the challenges and opportunities facing the blind spots in nanotoxicology in computationally dominated era by highlighting the future to accelerate nanomedicine clinical translation. As shown in Figure 1, an Artificial Intelligence (AI) and Machine Learning (ML) toolbox can immensely help in defining the core properties of nanomaterials relevant to biological activity, which will be useful for applications related to minimize toxicity in the context of nanotherapeutics. Because the bio‐physicochemical interface of the NP corona determines the biological identity of the NP, unlocking the material properties, size/shape, and surface characteristics using ML algorithms may reveal important insights into NPs and their interaction with the surrounding environment. Furthermore, NPcell interactions in vitro in viscous biological media with a high salt concentration act as another stealth layer of nanotoxicology. Here as well, AI approaches may help to decode the dissolution behavior, electrostatic agglomeration and accumulation, and the competitive binding of protein over NPs. These characteristics are generally complex and difficult to predict using conventional experimental methods based on a correlation of data or an identification of the most influential parameters. In particular, phase transformations and a free energy release based predictive relation can be established to decode the nano-bio interface of nanotoxicology using ML tools [38].
So, another research regarding to self-assembly of short amino acid sequences to design Metal-Biomolecule Frameworks (MBioFs) was done by Singh AV, et al. They reported a facile, bioinspired route of anisotropic nanostructure synthesis by using gold binding peptides (10-15mers) secreted by cancer cells. The bioinformatics tool-iterative Threading Assembly Refinement (i-TASSER) predicts the effect of amino acid sequences on metal binding sites and the secondary structures of the respective peptide sequence. Electron microscopy, X-ray, infrared, and Raman spectroscopy validated the versatile anisotropic gold nanostructures and the metal-bioorganic nature of biomineralization. They studied the influence of precursor salt, pH, and peptide concentration on the evolution of nanoleaf, nanoflower, nanofiber, and dendrimer-like anisotropic MBioFs. Characterization of photothermal properties using infrared laser (785 nm) revealed excellent conversion of light into heat. The result of their study revealed that exposure of bacterial cells in culture exhibits high rate of photothermal death using lower laser power (1.9 W/cm2) compared with recent reports. The MBioF’s self-assembly process can readily be extended and adapted to superior plasmonic material synthesis with a photothermal effect for in vivo biofilm destruction and cancer hyperthermia applications [39].
In an in vitro nanotoxicity system, cell-nanoparticle interaction leads to the surface adsorption, uptake, and changes into nuclei/ cell phenotype and chemistry, as an indicator of oxidative stress, genotoxicity, and carcinogenicity. In this regard, the study by Singh AV, et al. and his research team delineated the details of the Cell Shape Index (CSI) and Nuclear Area Factors (NAFs) as a descriptor of the type of nanomaterials. They proposed that the phenotypic variation in the epithelial cell is governed by the physicochemical descriptors (e.g., shape, size, zeta potential, concentration, diffusion coefficients, polydispersity, and so on) of the different classes of nanomaterials, which critically determines the intracellular uptake or cell membrane interactions when exposed to the epithelial cells at sub-lethal concentrations [40].
Anisotropic gold nanostructures are also attracting attention due to the strong correlation between their shape and the localized surface plasmon resonances, which allows tuning their optical responses through morphological optimizations [41].
Certainly, the field of nanomedicine needs several decades to develop. Perhaps before we allow our imagination to freely fantasize about the promises made by nanomedicine, it is better to consider capabilities that are truly feasible [5,6]. The story of nanotechnology in medicine will become the story of the development of surgical control at the molecular level and it will also create dramatic changes in the field of health and treatment. The use of nano robots or bio nano robots for drug delivery will reduce the side effects of drugs and the treatment will be performed faster; because only the patient’s tissue is subjected to medication [11,20].
In surgery nano robots can go to places in the body that humans and ordinary tools cannot reach [10]. It is likely that this technology will be used for treatment and early diagnosis of the disease, which is more important than treatment [2].
Nowadays, the widespread use of telemedicine makes it possible to decentralize the activities that are normally performed in the second level of health care, and this function can be provided at the level of primary care. Also, telemedicine can provide continuity and quality improvement in health care services. Now that the optimal use of resources is considered to be the most cost-effective activity for the economic aspects, this technology can be less costly than the usual activities [4].
Also, according to the existing operators on these new technologies, it is possible to provide the possibility of directing the operation of this equipment through telemedicine systems with some arrangements. Therefore, by designing integrated systems including the technological requirements of micro systems, nano-robotics and telemedicine systems will provide unique capabilities of this system in preventing, diagnosis, treatment and rehabilitation of remote patients. They can communicate wirelessly and remotely, and even on the move under normal living conditions [18]. Establish the benefits of these technologies and treat the potential risks in the form of special alarms for physicians in a timely manner. Treat patients in a timely manner and without delay. And sometimes uniquely remote can be well used as diagnostic methods in irreplaceable maneuvers in remote places and areas such as the arctic and antarctic space stations and similar places.
- Smrke A, Younger E, Wilson R, Husson O, Farag S, et al. (2020) Telemedicine during the COVID-19 pandemic: Impact on care for rare cancers. JCO Glob Oncol 6: 1046-1051. [Ref.]
- Pandya A, Auner G (2004) Robotics technology: a journey into the future. Urol Clin North Am 31: 793-800. [Ref.]
- Bhaskar S, Bradley S, Sakhamuri S, Chattu VK, Pandya S, et al. (2020) Designing futuristic telemedicine using artificial intelligence and robotics in the COVID-19 era. Front Public Health 8: 556789. [Ref.]
- Vandenberghe W, Moerman I, Demeester P (2012) Adoption of vehicular ad hoc networking protocols by networked robots. Wireless Pers Commun 64: 489-522. [Ref.]
- Li J, Esteban-Fernández de Ávila B, Gao W, Zhang L, Wang J (2017) Micro/nanorobots for biomedicine: Delivery, surgery, sensing, and detoxification. Sci Robot 2: eaam6431. [Ref.]
- Hollander JE, Carr BG (2020) Virtually perfect? Telemedicine for COVID-19. N Engl J Med 382: 1679-1681. [Ref.]
- Boggan JC, Shoup JP, Whited JD, Van Voorhees E, Gordon AM, et al. (2020) Effectiveness of acute care remote triage systems: A systematic review. J Gen Intern Med 35: 2136-2145. [Ref.]
- Klar M (2020) What is remote healthcare and how does it work? Advanced Data Systems. [Ref.]
- Mohamed KT, Ata AA, EI-Souhily BM (2011) Dynamic analysis algorithm for a micro-robot for surgical applications. Int J Mech Mater Des 7: 17-28. [Ref.]
- Mertz L (2018) Tiny conveyance: Micro- and nanorobots prepare to advance medicine. IEEE Pulse 9: 19-23. [Ref.]
- Arvidsson R, Hansen SF (2020) Environmental and health risks of nanorobots: an early review. Environ Sci: Nano 7: 2875-2886. [Ref.]
- Singh AV, Kishore V, Santomauro G, Yasa O, Bill J, et al. (2020) Mechanical coupling of puller and pusher active microswimmers influences motility. Langmuir 36: 5435-5443. [Ref.]
- Singh AV, Sitti M (2016) Patterned and specific attachment of bacteria on biohybrid bacteria-driven microswimmers. Adv Healthc Mater 5: 2325-2331. [Ref.]
- Singh AV, Ansari MHD, Mahajan M, Srivastava S, Kashyap S, et al. (2020) Sperm cell driven microrobots-emerging opportunities and challenges for biologically inspired robotic design. Micromachines (Basel) 11: 448. [Ref.]
- Singh AV, Sitti M (2016) Targeted drug delivery and imaging using mobile milli/microrobots: A promising future towards theranostic pharmaceutical design. Curr Pharm Des 22: 1418-1428. [Ref.]
- van der Meel R, Sulheim E, Shi Y, Kiessling F, Mulder WJM, et al. (2019) Smart cancer nanomedicine. Nat Nanotechnol 14: 1007- 1017. [Ref.]
- Lin L, Rasouli M, Kencana AP, Tan SL, Wong KJ, et al. (2011) Capsule endoscopy-A mechatronic perspective. Front Mech Eng 6: 33-39. [Ref.]
- Pan G, Wang L (2012) Swallowable wireless capsule endoscopy progress and technical challenges. Gastroenterol Res Pract 2012: 841691. [Ref.]
- Singh AV, Ansari MHD, Dayan CB, Giltinan J, Wang S, et al. (2019) Multifunctional magnetic hairbot for untethered osteogenesis, ultrasound contrast imaging and drug delivery. Biomater 219: 119394. [Ref.]
- Soto F, Wang J, Deshmukh S, Demirci U (2020) Reversible design of dynamic assemblies at small scales. Adv Intell Syst 2000193. [Ref.]
- Fabienne D, Véronique P (2015) Strategies to improve the EPR effect for the delivery of anti-cancer nanomedicines. Can Cell Microenviron 2: e808. [Ref.]
- Singh AV, Hosseinidoust Z, Park B, Yasa O, Sitti M (2017) Microemulsion-based soft bacteria-driven microswimmers for active cargo delivery. ACS Nano 11: 9759-9769. [Ref.]
- Irina Lyublinskaya, Gregg Wolfe, Douglas Ingram, Liza Pujji, Sudhi Oberoi, et al. (2015) Fluid dynamics and its biological and medical applications. In: College Physics for AP® Courses. College Physics, OpenStax, Houston, Texas. [Ref.]
- Singh AV, Batuwangala M, Mundra R, Mehta K, Patke S, et al. (2014) Biomineralized anisotropic gold microplate-macrophage interactions reveal frustrated phagocytosis-like phenomenon: A novel paclitaxel drug delivery vehicle. ACS Appl Mater Interfaces 6: 14679-14689. [Ref.]
- Singh AV, Jungnickel H, Leibrock L, Tentschert J, Reichardt P, et al. (2020) ToF-SIMS 3D imaging unveils important insights on the cellular microenvironment during biomineralization of gold nanostructures. Sci Rep 10: 261. [Ref.]
- Fratila RM, Rivera-Fernándeza S, de la Fuente JM (2015) Shape matters: Synthesis and biomedical applications of high aspect ratio magnetic nanomaterials. Nanoscale. 7: 8233-8260. [Ref.]
- Khandel P, Yadaw RK, Soni DK, Kanwar L, Shahi SK (2018) Biogenesis of metal nanoparticles and their pharmacological applications: present status and application prospects. J Nanostruct Chem 8: 217-254. [Ref.]
- Meyer T, Venus T, Sieg H, Böhmert L, Kunz BM, et al. (2019) Simultaneous quantification and visualization of titanium dioxide nanomaterial uptake at the single cell level in an in vitro model of the human small intestine. Small Methods 3: 1800540. [Ref.]
- Song R, Murphy M, Li C, Ting K, Soo C, et al. (2018) Current development of biodegradable polymeric materials for biomedical applications. Drug Des Devel Ther 12: 3117-3145. [Ref.]
- Singh V, Kumar V, Kashyap S, Singh AV, Kishore V, et al. (2019) Graphene oxide synergistically enhances antibiotic efficacy in vancomycin-resistant Staphylococcus aureus. ACS Appl Bio Mater 2: 1148-1157. [Ref.]
- Nummelin S, Shen B, Piskunen P, Liu Q, Kostiainen MA, et al. (2020) Robotic DNA Nanostructures. ACS Synth Biol 9: 1923-1940. [Ref.]
- Walker D, Käsdorf BT, Jeong HH, Lieleg O, Fischer P (2015) Enzymatically active biomimetic micropropellers for the penetration of mucin gels. Sci Adv 1: e1500501. [Ref.]
- Lipfert J, Doniach S, Das R, Herschlag D (2014) Understanding nucleic acid-ion interactions. Annu Rev Biochem 83: 813-841. [Ref.]
- Helmholtz Association of German Research Centres (2011) Water molecules characterize the structure of DNA genetic material. ScienceDaily. [Ref.]
- Singh AV, Ansari MHD, Laux P, Luch A (2019) Micro-nanorobots: important considerations when developing novel drug delivery platforms. Expert Opin Drug Deliv 16: 1259-1275. [Ref.]
- Moore R (2013) Unlocking the potential of medical nanotechnology. Eur Med Dev Technol 4.
- Sweeney AE (2015) Nanomedicine concepts in the general medical curriculum: initiating a discussion. Int J Nanomedicine 10: 7319- 7331. [Ref.]
- Singh AV, Rosenkranz D, Ansari MHD, Singh R, Kanase A, et al. (2020) Artificial intelligence and machine learning empower advanced biomedical material design to toxicity prediction. Adv Intellig Sys 2: 2000084. [Ref.]
- Singh AV, Jahnke T, Wang S, Xiao Y, Alapan Y, et al. (2018) Anisotropic gold nanostructures: optimization via in silico modeling for hyperthermia. ACS Appl Nano Mater 1: 6205-6216. [Ref.]
- Singh AV, Maharjan RS, Kanase A, Siewert K, Rosenkranz D, et al. (2021) Machine-learning-based approach to decode the influence of nanomaterial properties on their interaction with cells. ACS Appl Mater Interfaces 13: 1943-1955. [Ref.]
- Piotto V, Litti L, Meneghetti M (2020) Synthesis and shape manipulation of anisotropic gold nanoparticles by laser ablation in solution. J Physic Chem 124: 4820-4826. [Ref.]
Download Provisional PDF Here
Article Type: REVIEW ARTICLE
Citation: Shahri SSZ, Sayyedalhosseini S (2021) Use of Medical Micro and Nano Robots in Telemedicine in COVID-19 Pandemic. Int J Nanomed Nanosurg 5(1): dx.doi.org/10.16966/2470-3206.125
Copyright: © 2021 Shahri SSZ, et al. This is an open-access article distributed under the terms of the Creative Commons Attribution License, which permits unrestricted use, distribution, and reproduction in any medium, provided the original author and source are credited.
Publication history:
SCI FORSCHEN JOURNALS
All Sci Forschen Journals are Open Access