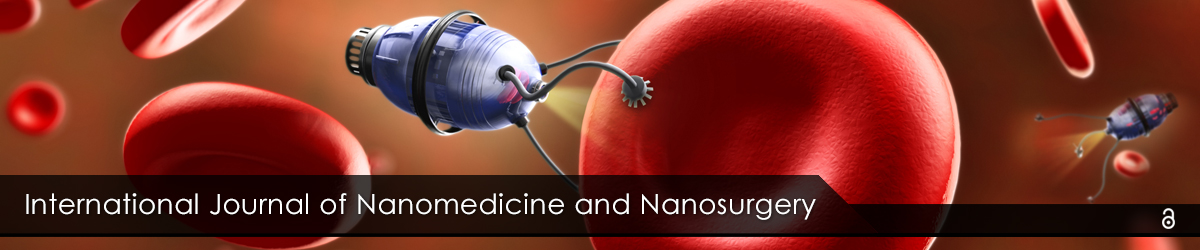
Full Text
Nanotechnology and the Applications in Life Science
Costas A. Charitidis*
Corresponding author: Costas A. Charitidis, Research Unit of Advanced, Composite, Nano-Materials and Nanotechnology, School of Chemical Engineering, National Technical University of Athens, Greece. E-mail: charitidis@chemeng.ntua.gr
Editorial Overview:
Over the past decade, nanotechnology has been used in many different fields of science as an innovative tool for technological evolution. Nanotechnology is a field of technology that brings in close collaboration many scientists such as physicists, chemists, mathematicians, biologists, doctors and engineers in order to expand the research mentality across the traditional disciplinary boundaries. The vision of nanotechnology focuses on various societal goals, such as improved comprehension of nature, increased productivity, improved health care and higher limits of sustainable development and human potential [1]. Nanotechnology can be defined as the technology that emphasizes on the design, characterization, production and application of structures, devices and systems that present unique physical, chemical and biological properties by controlling size and shape of nanomaterials. On the contrary, nanoscience refers to the study of phenomena and the manipulation of matter at the atomic and molecular level, where significant differences in matter properties are recorded compared to bulk materials. The orientation of nanotechnology in life sciences has led to the evolution of nanomedicine that is considered as a subdiscipline contained within nanotechnology and nanoscience.
This evolution has led to new materials and equipment that significantly improve the quality of life, provide targeted therapy and innovative therapies, ultimately, aiming in the future performance of surgeries using nanorobots. Hence, nanomedicine and bionanotechnology have attracted tremendous attention due to the promising medical application of nanotechnology and the decrease of side effects observed till now at the limited numbered in vivo and trial tests performed. However, there is still much effort to be put in order to fully approve nanomedical materials, devices and nanorobots and reassure the future use for the well-being. Nevertheless, many legal and ethical committees are addressing the issue of safety and applicability of engineered nanomaterials in life science and are continuously setting new boundaries for the characterization and testing of nanomaterials.
The past decades different kinds of nanomaterials have been synthesized for biomedical application. The most widely studied class of materials is oxide nanoparticles and most importantly superparamagnetic iron oxides nanoparticles that have been used in cell separation, as imaging contrast agents and diagnostic tools, for targeted drug delivery and gene transfection, hyperthermia therapy and many other applications. Another class is gold nanoparticles that are mostly used for imaging applications of cancer sites. More recently, scientists developed mesoporous silica nanoparticles that can be used as drug, proteins and/or nucleotides carriers [2-4], due to the advantages obtained from the formed pore cavities. Additionally, research has been conducted in the synthesis of polymer-based soft nanoparticles, i.e. dendrimers and/or block-copolymers, for the delivery of DNA, proteins and peptides [5-8]. Apart from the spherical shaped particles attention has been given to other shaped particles for the delivery of DNA and drugs such as nanorods [9] and nanotubes [10]. Lately, it has been shown that carbon nanotubes can be used in drug delivery systems for cancer therapy [11], as well as in imaging, DNA delivery in living cells and tissue engineering applications [12]. However, as already stated limited research has been conducted in the evaluation of in vitro and in vivo activity of nanomaterials, in order to assess their toxicity effect and most importantly their efficiency in real condition experiments [13,14].
Scientists now are designing and synthesizing nanomaterials that present complex engineered surface characteristics and can be externally or internally triggered by different stimuli such as pH, heat, light, sound, and mechanical or magnetic force. Consequently, these nanomaterials can achieve targeting on malignant tissues, progressively drug or gene release or even act as enhancement tools in already widely used diagnostic methods. The new proposed materials are firstly studied in respect of their release and pharmacokinetic behavior in flow and the experimental results are simulated with suitable mathematical equations for evaluating their actual motion in organisms. These simulation models are quite interesting in cases such as atherosclerosis, where stenosis can prohibit flow and ultimately, targeted delivery of smart nanoparticles. Now-a-days scientists apart from the synthesis of nanoparticles for drug delivery and/ or diagnostic applications have also focused on the development of smart coatings onto coronary arterial drug-eluting stents for promoting drug release in the local area of stenosis, or avoiding the absorption of cells and proteins onto the stent that will eventually result in the failure of the stent [15-18].
Other materials that are offering low-cost options for disease diagnosis are the microfluidic and nanoelectronic devices that have been developed [19]. All these devices provide accurate results in short time, significantly helping in treatment. The future though will lie in the development of smart devices or patches that will enable health monitoring of human beings, store the information and deliver a treatment if necessary. Additionally, nanomedicine apart from nanomedical materials and devices contains the research performed in nanoscale surgery and tissue engineering. Nanoscale surgery or nanosurgery is the replacement of traditional surgical instruments by nanotechniques in order to achieve the best outcome in the level of individual living cells or organelles. Consequently, nanoscale techniques such as atomic force microscopes (AFM) equipped with nanoneedles or tips manufactured by a single carbon nanotube structure or femtosecond lasers can achieve localized treatment limiting also the damage caused to the surrounding healthy cells or tissue. In the same perspective lasers can aid in gene or cell therapy and even induce cells differentiation in tissue engineering applications. Furthermore, nanotechnology has assisted in the development of suitable devices and techniques that can provide useful information on the malignant or nonmalignant state of tissues and living cells.
Various already used nanotechniques can be further improved and in the future can be employed as diagnostic tools in order to identify malignant and non-malignant tissues, or interaction of living cells with the surrounding environment. Nanobiomechanics are the technology applied for the understanding of the fundamental behaviour of living cells and biomolecules. Through nanoscale investigation of mechanical properties of living cells, the mechanical interaction of cells with their surrounding structures, as well as with other cells, is assessed [20]. This is considered crucial, particularly when trying to develop new tissue engineering and regenerative medicine strategies. For example, measuring nanoscale forces exerted by proteins on cells can potentially afford new understanding on various diseases, such as osteoarthritis [21], cancer and malaria [22] due to cells elasticity and adhesion alterations. Cells, when interacting with the surrounding extracellural matrix or with biomaterials (scaffolds, coatings and bulk materials), are greatly affected, potentially influencing the process of mechanical signal transduction in living tissues [23-25].Consequently, nanobiomehanics needs the equal contribution of doctors, biologists, engineers, physicists and mathematicians in order to assess all the biological processes, improve instruments and assess the experimental results. All the obtained information from the single cell analysis will benefit towards the biological understanding of disease initiation and progression, the development of improved diagnostic tools and improvement or development of innovative therapeutic approaches.
References
- Roco MC (2003) Broader societal issues of nanotechnology. J Nanopart Res, 2003, 5: 181–189.[Ref.]
- Galbraith DW (2007) Nanobiotechnology: silica breaks through in plants. Nat Nanotechnol, 2: 272 – 273.[Ref.]
- Li X, Zhang J, Gu H (2011) Adsorption and Desorption Behaviors of DNA with Magnetic Mesoporous Silica Nanoparticles. Langmuir, 27: 6099-6106.[Ref.]
- Mackowiak SA, Schmidt A, Weiss V, Argyo C, von Schirnding C, et al. (2013) Targeted drug delivery in cancer cells with red-light photoactivated mesoporous silica nanoparticles. Nano Letters, 13: 2576–2583.[Ref.]
- Cheng Z , Elias DR , Kamat NP , Johnston ED, Poukhtine A, et al. (2011) Improved Tumor Targeting of Polymer-based Nanovesicles Using Polymer-Lipid Blends. Bioconjugate Chem, 22: 2021-2029.[Ref.]
- Hu Y, Haynes MT, Wang Y, Liu F, Huang L (2013) A highly efficient synthetic vector: nonhydrodynamic delivery of DNA to hepatocyte nuclei in vivo. ACS Nano7: 5376 – 5384.[Ref.]
- Navarro G, Movassaghian S, Torchilin PV (2013) Current Trend in the Use of Cationic Polymer Assemblies for siRNA and Plasmid DNA Delivery. Pharmaceutical Nanotechnology, 1: 165- 183.[Ref.]
- Simeon RL, Chamoun AM, McMillin T, Chen Z (2013) Discovery and Characterization of a New Cell-Penetrating Protein. ACS Chemical Biology, 8: 2678-2687.[Ref.]
- Ramos J, Huang HC, Rege K (2013) Delivery of Plasmid DNA to Mammalian Cells Using Polymer–Gold Nanorod Assemblies. Cellular and Subcellular Nanotechnology: Methods in Molecular Biology, 991: 81-91. [Ref.]
- Li J1, Fan C, Pei H, Shi J, Huang Q (2013) Smart Drug Delivery Nanocarriers with Self-Assembled DNA Nanostructures. Adv Mater. 25: 4386-4396.[Ref.]
- Zhang W, Zhang Z, Zhang Y, (2011) The application of carbon nanotubes in target drug delivery systems for cancer therapies. Nanoscale ResLett, 6:555.[Ref.]
- Liu Z, Tabakman S, Welsher K, Dai H (2009) Carbon Nanotubes in Biology and Medicine: In vitro and in vivo Detection, Imaging and Drug Delivery. Nano Research, 2: 85-120.[Ref.]
- Díaz JA, Gibbs-Davis JM, (2013) Sharpening the thermal release of DNA from nanoparticles: towards a sequential release strategy. Small, 9: 2862-2871.[Ref.]
- Suna H, Zhu X, Zhang L, Zhang Y, Wang D (2010) Capture and release of genomic DNA by PEI modified Fe3O4/Au nanoparticles. Materials Science and Engineering C. 30: 311–315.[Ref.]
- Carlyle WC, McClain JB, Tzafriri AR, Bailey L, Zani BG, et al. (2012) Enhanced drug delivery capabilities from stents coated with absorbable polymer and crystalline drug. J Control Release, 162: 561-567.[Ref.]
- Holmes DR Jr (2003) State of the art in coronary intervention. Am J Cardiol, 91: 50A-53A.[Ref.]
- Gunn J, Cumberland D (1999) Stent coatings and local drug delivery: State of the art. Eur Heart J, 20: 1693-1700.[Ref.]
- Kastrati A1, Mehilli J, Dirschinger J, Dotzer F, Schühlen H et al. (2001) Intracoronary stenting and angiographic results: strut thickness effect on restenosis outcome (ISAR-STEREO) trial. Circulation, 103: 2816-21.[Ref.]
- Morris JE, Iniewski K (2013) Nanoelectronic Device Applications Handbook (Devices, Circuits, and Systems). Boca Rotan : CRC Press Taylor & Francis Group.[Ref.]
- Liu KK, Oyen ML (2014) Nanobiomechanics of living materials. Interface Focus, 4: 20140001.[Ref.]
- Stolz M, Gottardi R, Raiteri R, Miot S, Martin I et al. (2009) Early detection of aging cartilage and osteoarthritis in mice and patient samples using atomic force microscopy. Nat Nanotechnol, 4:186-192.[Ref.]
- Lee GY, Lim CT (2007) Biomechanics approaches to studying human diseases. Trends Biotechnol, 25: 111-118.[Ref.]
- Liedert A, Kaspar D, Claes L, Ignatius A (2006) Signal transduction pathways involved in mechanical regulation of HB-GAM expression in osteoblastic cells. Biochem Biophys Res Commun, 342: 1070-1076.[Ref.]
- Robling AG, Bellido T, Turner CH (2006) Mechanical stimulation in vivo reduces osteocyte expression of selerostin. J Musculoskelet Neuronal Interact, 6: 354-354.[Ref.]
- Setton LA, Chen J (2006) Mechanobiology of the intervertebral disc and relevance to disc degeneration. J Bone Joint Surg Am, 88:52-57.[Ref.]
Download Provisional pdf here
Article Information
Article Type: Editorial
Citation: Costas A. Charitidis (2015) Nanotechnology and the Applications in Life Science. Int J Nanomed Nanosurg, Volume1.1: http://dx.doi.org/10.16966/ 2470-3206.e101
Copyright: © 2015 Charitidis CA. This is an open-access article distributed under the terms of the Creative Commons Attribution License, which permits unrestricted use, distribution, and reproduction in any medium, provided the original author and source are credited.
Publication history:
SCI FORSCHEN JOURNALS
All Sci Forschen Journals are Open Access