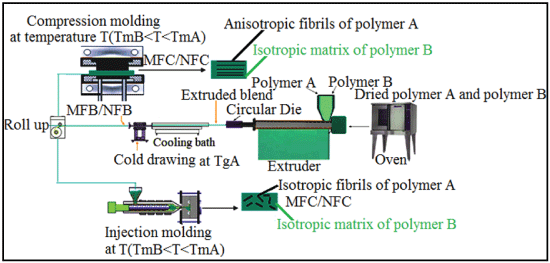
Figure 1: Scheme for preparation of in-situ micro/nanofibrillar reinforced polymer composites (MFC/NFC)-TmA: melting point of polymer A; TmB: melting point of polymer B; TmA>TmB; TgA: glass transition temperature of polymer A.
Raghvendra Kumar Mishra*
Indian Institute of Space Science and Technology, Valiamala, Thiruvananthapuram, Kerala, India*Corresponding author: Raghvendra Kumar Mishra, Indian Institute of Space Science and Technology, Valiamala Road, Valiamala, Thiruvananthapuram, Kerala 695547, India, Tel: 00-34-913941820; E-mail: raghvendramishra4489@gmail.com
This short article is an attempt to collect information and facts from the team of globally recognized scientists as well as experts and also deliver the perspective of in-situ reinforced microfibrillar composites. It is well established that melt-spinning or even solidstate stretching (drawing) of polymers creates high-modulus, high-strength polymer fibres. In-situ microfibrillar reinforced composites (MFCs) or nanofibrillar reinforced composites (NFCs) are impressive systems, which are created from melt blendingcold/hot drawing followed by compression or injection moulding of immiscible plastic blends. For example, Poly (propylene)/ Poly (ethylene terephthalate), Poly (propylene)/Nylon-6, Poly (propylene)/Nylon-66, Poly (propylene)/Polycarbonate, Poly (ethylene)/Poly (ethylene terephthalate), Poly (ethylene)/ Nylon-66, Polyethylene/Polycarbonate, Poly (ɛ-caprolactone) (PCL)/Poly (lactic acid) (PLA) are widely used blends systems for the MFC. This review article focuses on the improvement of properties of aforesaid blends systems by means of the in-situ reinforced micro/nanofibrillar composite principle.
Plastic blend; Plastic; In-situ reinforced microfibrillar composites; Biomedical; Application; Materials
In-situ microfibrillar reinforced composites (MFCs) or nanofibrillar reinforced composites (NFCs) based on two thermoplastics has gained a lot of interest because this type of material provides a way to tailor the properties of an immiscible plastic blends for the desired application. An in–situ microfibrillar reinforced composites (MFCs) or nanofibrillar reinforced composites (NFCs) comprises of a pair of thermoplastic polymers having an independent as well as the difference in their melting point temperatures. This type of in–situ microfibrillar reinforced composites (MFCs) or nanofibrillar reinforced composites (NFCs) is usually produced by the three main processing protocols [1]: (1) Melt blending of the virgin plastics (having distinct melting point TmB<TmA) and afterwards extrusion, (2) Cold stretching (drawing) of the blend at glass transition temperature (Tg) of the higher melting point polymer (TmA), called as microfibrillar blend (MFB) or nanofibrillar blend (NFB), (3) The successive annealing (through compression or injection molding) of the microfibrillar blend (MFB) or nanofibrillar blend (NFB) at fixed temperature (T) (TmB<T<TmA), in which TmA and TmB are the melting temperatures of the two polymers, respectively. After drawing, the polymers in the blend are completely seen to be oriented in the form of micro/ nanofibrils with average diameters of around μm to nm rages, so termed an in-situ micro/nanofibrillar blend (MFB/NFB). The Micro/Nanofibrillar blend (MFB/NFB) is transformed into in-situ reinforced micro/nanofibrillar composites (MFC/NFC) through compression or injection moulding at temperature (T), resultant, higher melting point polymer act as reinforcement in the form of the fibrils and lower melting point polymer act as a matrix [2], as shown in Figure 1.
Figure 1: Scheme for preparation of in-situ micro/nanofibrillar reinforced polymer composites (MFC/NFC)-TmA: melting point of polymer A; TmB: melting point of polymer B; TmA>TmB; TgA: glass transition temperature of polymer A.
Additional improvement in the idea of microfibrillar blend (MFB) or nanofibrillar blend (NFB) led to the production of a numerous kind of MFC or NFC from various plastic blends. For example, making use of the particular solvent for extraction of the matrix has been well explained, resultant, the micro/nanofibrillar can be used for production of fabrics [3]. It is confirmed that the in-situ reinforcing micro/nanofibrils are isolated as an individual material from in-situ reinforced micro/nanofirbillar composites, and also can be used for the various application. The in-situ reinforced micro/nanofirbillar composites idea permits to convert many kinds of virgin or waste plastics into a fibrillar nanomaterial, additional processing can be used as per technological as well as particular requirements [4]. Considerably, more tricky application appears to be their biomedical application, for example, in the area of regenerative drugs and medical science. The requirement for scaffold materials for tissue architecture and engineering is increasing [5]. Looking at the reality that overall performance of the scaffold is dependent upon the surface of the scaffolding materials, nanofibrils are an outstanding choice to do this task. In addition, the in-situ reinforced micro/nanofirbillar composites strategy can be employed to biodegradable as well as biocompatible polymers. These kinds of composites may also be used in role of carriers for regulated drug delivery. For this specific purpose, the fibrils must have a rough surface (as well as much better be porous) to be able to improve the drug loading abilities. More sensibly, the fine sizes and the significant surface area in relation to the volume, which is accessible on a nanofibril for medicine loading, and allow it to be a reasonable choice [6]. To improve the roughness, various types of nanoparticles can also be used along with the immiscible plastics blends.
The benefits of the polymer-polymer composites with MFC or even NFC configuration which have been produced and it consist the following advantages: strengthen of polymer by polymer, absolutely no mineral ingredients, simple handling, lightweight, synergetic impact in mechanical characteristics, entire reusable as well as recyclable, excellent distribution of nanofibrils in NFC (hardly any aggregates), opportunities for isolation of micro or nanofibrils, without having of additional compatibilizers (for condensation polymers, it offers self compatiblizing effect) [6]. That means, whenever the blend consists of a pair of condensation polymers, a selfcompatibilization effect can also be analysed as a result of the interchange chemical reactions leading to the creation of a copolymer at the phase boundaries. The mechanical characteristics of MFC/NFC are near to those of commercially produced short glass fibre-reinforced thermoplastics with the same matrix. MFC/NFC including polyolefins, for example, polypropylene (PP) or even polyethylene in the form of matrices, and poly (ethylene terephthalate) in the role of reinforcement exhibit the identical morphological. Also, structural features MFC/NFC based on condensation polymers including PET and polyamides have already been investigated. Their mechanical properties (e.g. tensile strength in addition to Young’s modulus) are five times greater in comparison to the virgin PP and PE matrices. In comparison with short glass fibre-reinforced composites (30 wt% glass fibres), possessing the similar matrix (LDPE), the MFC/NFC provide roughly the equivalent Young’s modulus and tensile strength along with significantly better (around 10 times) deformation potential. In-situ reinforced binary as well as ternary polymer/polymer composites are produced by the melt mixing of poly (ethylene terephthalate) (PET), polyamide-6 (PA-6), and polyamide-66 (PA-66) within an extruder in the appearance of a catalyst , and then stretching (drawing) of the extrudate as well as annealing of the drawn blends. The existence of chemical bonds between the individual polymer through copolymers leads to the co crystallization of PA-66 as well as PA-6 as well as the supportive crystallization of PET, PA-6, and PA-66, promoting the compatibility of the blend components [7].
Reused poly (ethylene terephthalate) from waste poly (ethylene terephthalate) containers and bottles has been employed in the role of a strengthening material for isotactic polypropylene related to the thought of in-situ-reinforced microfibrillar composites. Microfibers of PET have been effectively produced during the period of melt-extrusion and succeeding drawing and consequently maintained in the concluding injection-moulded specimens. The consequences of draw ratio, preliminary dimensions of ground PET flakes, and PET proportion on the morphology of the extrudates along with them as-produced PET fibres and mechanical features of the as-prepared MFC have been examined. Flexural moduli, tensile modulus, as well as the tensile strength of MFC are enhanced by the existence of PET microfibers and additionally, enhancement is accomplished by the inclusion of maleic anhydride-grafted isotactic polypropylene that has been applied just as the compatibilizer [8].
It has been carried out to create an alternative conductive carbon black (CB) filled poly (ethylene terephthalate) (PET)/ polyethylene (PE) in-situ microfibrillar composites with an inferior percolation threshold via selectively localizing CB particles in the surfaces of the PET microfibrils. The CB particles were initially blended with PE matrix, after which PET was included into CB/PE mixture. Consequently, the CB/PET/PE composite was exposed to a slit die extrusion, hot stretch as well as quenching technique to produce in-situ PET microfibrils, wherein CB particles migrated to the surfaces of the PET microfibrils at the same time. The morphological viewpoint revealed that the PET phases produced definite microfibrils, and CB particles have done overwhelmingly localize in the surfaces of the PET microfibrils, which contributed to an extremely low percolation threshold, i.e. 3.8 vol%, and a reliable conductivity. The conductive network is developed by the interaction as well as overlapping of the CB particles covered PET microfibrils. Additionally, the CB particles staying in the PE matrix additionally played a major role in the conductive paths, particularly for the high CB loading incorporated microfibrillar composites [7].
The solvent and gas barrier properties are improved by the micro/nanofibrils. The in-situ generation fibrils act as nucleating agent for thermal crystallization and the trans crystallites only occur in in-situ reinforced micro/nanofibrillar composites [4,9-13]. Therefore, the processing, as well as functioning of MFC, is effectively noticed on industrial size products. MFC receive high-quality practical application possibilities in the automobile construction because they are free from mineral reinforcement. The isolation of nano- as well as microfibrils by means of particular extraction of the second blend component (matrix), provides opportunities for their biomedical uses such as scaffolds for the regenerative drugs or even as carriers for regulated medicine delivery together with nano filter materials [4,10-13].
Biodegradable polymers have satisfied with a rising interest in biomedical application throughout the last decades. Without a doubt one of these kinds of polymers is poly (ε-caprolactone) (PCL) that is polyester which has been commonly employed in tissue engineering area for its accessibility, comparatively cheap price level as well as convenience for modification. Its chemical together with biological characteristics, physicochemical condition, degradability as well as mechanical durability is generally modified, thereby; it is usually applied under severe mechanical, physical and chemical environment without major decay in its features. Degradation time period of PCL is pretty lengthy; as a result, it is employed primarily in the replacement of hard tissues in the body in which recovery possibly requires a prolonged time period. Additionally, it is employed in loadbearing tissues of the body by improving its stiffness [14]. Nevertheless, because of its tailorability, application of PCL is not limited to one kind of tissue also it is widespread to the engineering of soft tissues by lowering its molecular weight together with degradation time. This part of review describes the fundamental characteristics of PCL, its in-situ reinforced micro/nano-fibrillar composites for the healthcare applications for example tissue engineering as well as medicine delivery systems. Poly (e-caprolactone) is a biodegradable material with lots of beneficial characteristics for biomedical, product and food packaging applications. Nevertheless, its application is restricted by lower yield stress, stiffness. Consequently, it has remarkably ductile deformation under the ambient situation. The main factor is the low glass transition temperature as well as limited stable crystalline configuration. Mechanical performance is modified by mixing, the inclusion of an appropriate biodegradable polymer to protect biodegradability. Usually, the possibility of enhancing mechanical characteristics of PCL by means of blending is comparatively low [6,15]. An additional procedure for improving PCL mechanical performance is the production of composites having PLAbased fibers, mostly produced by using electrospinning. The best-suited strategy is being investigated the influence of in-situ developed the melt-drawn fibrillar structure of Poly (lactic acid) (PLA) in the PCL matrix. In consideration that the injection-molding of the microfibrillar blend were handled at the temperature (T), which is below the melting temperature of the PLA fibrils, and finally, the in-situ PLA micro/nanofibrillar reinforced composite with the PCL matrix was obtained. As per processing methods of the in-situ microfibrillar composites, two biodegradable plastics PLA (Pa) and PCL (Pb) (Pa and Pb having different melting point temperature, melting point temperature and glass transition temperature of Pa>melting point and glass transition temperature of Pb) are dried out at in a vacuum oven. The blending together of PLA (Pa) and PCL (Pb) is performed in a counter-rotating single screw or twin-screw extruder. The extruded bristle Pa/Pb extruded is melt/cold drawn by making use of a drawing unit to transform the extruded blend into the micro/nanofibrillar blend. Microfibrillar blend is changed into in-situ micro/nanofibrillar reinforced composites through compression/injection molding. The MFC/NFC structures are known by an isotropic matrix, which has reinforcing fibrils of isotropic orientation after injection molding or even of anisotropically orientation in the case of compression molding [16,17]. The weight ratio of the polymer (Pa) and Polymer (Pb) and also injection/compression molding profoundly changes the morphology as well as properties of the in-situ micro/ nanofibrillar-reinforced composite. The expected morphology of in-situ micro/nanofibrillar reinforced composites is shown in Figure 2.
Figure 2: Morphology development of in-situ micro/nanofibrillar reinforced composites: Step 1) Spherical morphology of polymers Pa and Pb after melt blending; Step 2) Elliptical morphology of polymers Pa and Pb after extrusion; Step 3) Fibrils morphology of the (Pa) and (Pb) after cold/hot drawing; Step 4) a) Anisotropically distributed fibrils of the Polymer (Pa) within the isotropic matrix of the polymer (Pb) after the compression molding, b) Isotropically distributed fibrils of the Polymer (Pa) in the isotropic matrix of the polymer (Pb) after the injection molding.
An innovative idea of producing micro/nanofibrillar reinforced composites (MFC/NFC) from polymer blends is stated. There are fundamentally three steps included in the production of polymer/polymer MFCs, viz: (a) blending of the component polymers, commonly in an extruder, (b) drawing or orientation and (c) annealing or the isotropization step. In the primary step, melt-blending of two or more immiscible thermoplastic polymers manifest with or without the presence of a compatibilizer. In the subsequent step, the extruded blend is either one cold drawn or hot drawn to accomplish fibrillar morphology of the polymers. In the next step, the drawn blends are annealed or isotropized which banishes the fibrillar morphology of the polymer with lesser melting point temperature (Tm). The fibrils of the polymer with higher melting point temperature (Tm) are maintained as they are isotropically or anisotropically distributed in an isotropic matrix of the polymer of the lower Tm. Because the matrix and the reinforcement are thermoplastic polymers as well as the reinforcements (fibrils of the polymer with the greater Tm) are produced in-situ throughout the procedure these are defined as in-situ reinforced polymer/polymer composites. When considering mass manufacturing of microfibrillar composites, the processing criteria must be fully optimized to resolve the chopping issue of the drawn strands along with the loading issue in the course of compression/injection molding. The significant difference in the thermal expansion coefficient, as well as Poisson number of various plastics pairs, may also be responsible for inadequate interfacial adhesion. Accordingly, it is essential to establish an approach for enhancing the interfacial adhesion that will contribute to additional enhancement in mechanical characteristics of the MFC/NFC. It is achievable and fascinating to receive miro/nanofibrils by changing the extrusion and stretching criteria. The creation of ranges of nanofibrils reinforced composites may open a meaningful outlook for improving the material characteristics. We need to consider various other biodegradable polymer blends as well as compatibilizers for generating new forms of MFC/ NFC. Considerably more beneficial is to use these particular nanofillers such as carbon nanotubes and carbon nanofibers which align independently throughout drawing (stretching) process.
Download Provisional PDF Here
Article Type: MINI REVIEW
Citation: Mishra RK (2018) Efficient In-Situ Reinforced Micro/ Nano Fibrillar Polymer-Polymer Composites: A New Class of Materials for Biomedical Application. J Med Chem Drug Des 1(1): dx.doi.org/10.16966/2578-9589.106
Copyright: © 2018 Mishra RK. This is an open-access article distributed under the terms of the Creative Commons Attribution License, which permits unrestricted use, distribution, and reproduction in any medium, provided the original author and source are credited.
Publication history:
All Sci Forschen Journals are Open Access