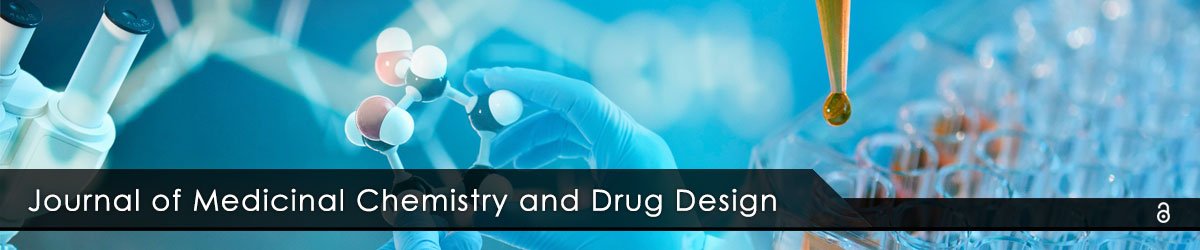
Full Text
Ayten Saracoglu1* Sermin Tetik2
1Department of Anesthesiology and Intensive Care, Istanbul Marmara University School of Medicine, Turkey2Department of Biochemistry, Istanbul Marmara University School of Medicine, Turkey
*Corresponding author: Saracoglu A, Department of Anesthesiology and Intensive Care, Medical Faculty of Istanbul Marmara University, Fevzi Cakmak Mh, Muhsin Yazicioglu Cd, No: 10 Pendik, Istanbul, Turkey, Tel: +90 216 657 06 06, E-mail: anesthesiayten@gmail.com
Platelets play a key role for the maintenance of hemostasis by interacting with endothelium and inflammatory pathways. In the presence of oxidative and nitrative stress, oxidative reactions lead to the formation of highly reactive ROS/RNS compounds. Those play a major role in controlling not only the activity but also the fate of platelets. The changed redox state of the platelets is also associated with increased formation or release of ROS/RNS in the vascular region, platelet activation and thrombotic risk. In the presence of trauma, inflammation and hypoperfusion results in the release of ROS by the cells such as platelets, leukocytes and endothelial cells causing major changes on coagulation via oxidation of PAI-1, protein C and thrombomodulin. In this review, the association between reactive oxygen species and platelet reveals in oxidative stress, inflammation and trauma.
Platelets (PLTs) are seedless and discoid-shaped blood cells, with a normal blood concentration of 150-350×109 /L. Platelets have a three-layer membrane structure, and the outer membrane is responsible for activation of surface cell receptors. The medium membrane reacts with adhesive proteins while the innermost sub-membrane layer controls signaling. Platelets have a typical diameter of 2–3 μm, and their average lifespan is approximately 9-11 days. There are microtubules and microfilaments on the skeleton of the platelets. The alpha granules in PLTs contain albumin, factor V, fibrinogen, fibronectin (FN), platelet factor 4 (PF4), von Willebrand factor (vWF), P-selectin and C1-esterase inhibitors, the compounds that increase adhesion, promote cellular interactions and trigger vessel repair. Dense granules contain serotonin, adenine nucleotide and calcium. Lysosomal enzymes contribute to the formation of blood clots. Platelets are derived from the megakaryocytes of the bone marrow, and their principal function is to prevent bleeding by forming a plug in the coagulation cascade. Under normal conditions, platelets play a role in the maintenance of hemostasis and also interact with endothelium, leukocytes and inflammatory pathways to preserve vascular integrity and vascular tone. The platelet activation cascade is, therefore, under a tight control. Disruption of this control may lead to problems such as atherosclerosis and carcinogenesis. For this reason, platelet activation is considered to be a good indicator of trauma, cancer, infection and coagulation disorders [1]. In this review we aimed to determine the association between reactive oxygen species and platelets in oxidative stress, inflamation and trauma.
Platelets and Hemostasis
Well known PLT regulatory factors include nitric oxide (NO), prostacyclin (PGI2 ), adenosine, and reactive oxygen species (ROS). If we need to examine hemostasis in phases, PLTs form the first phase of cellular hemostasis while the regulator factors play a role in the second phase and fibrinolysis comes into play in the last phase. As mentioned previously, platelets account for the initiation of the hemostasis process. The first phase of hemostasis is the initiation phase. In case of trauma, the tissue factor (TF) occurs with tissue damage and binds to FVII which activates Complex FX and Complex V. In this way, prothrombin is converted to thrombin activating PLTs [2].
The second phase is the amplification and activation phase. The PLTs in the injured area bind to released fibrinogen and become activated through skeleton transformation. Thrombin separates vWF from its complex with FVIII, and thus, FVIII becomes active. The third and the last phase is the propagation phase. FVIII combines with FIX to form a tenase complex, and FIX diffuses on the surface of PLT. Then, FVa and FXa connected to PLT membrane couple and generate a prothrombinase complex. Thus, a large amount of thrombin is produced from prothrombin which in turn ensures the conversion of fibrinogen to fibrin.
Signal transduction of platelets is achieved by glycoprotein Ib-V-IX (GPIb-V-IX), integrin aIIb b3, immunoglobulinfamily collagen receptor, GPVI, adhesive collagen receptor, integrin a2b1. Following the activation, platelets adheres to vWF generated in subendothelial matrix of GPIb/V/IX, leading to platelet capture at this specific phase of hemostasis.
GPVI mediates the activation and adhesion of platelets and binds the fibrinogen to collagen. The activation of PLTs with the GPVI, which forms a complex with the Fc receptor gammachain (FcRg), is provided through scaffold proteins such as LAT and Cbl-b, protein tyrosine kinase, phosphatidylinositol 3-kinase (PI 3-kinases) as well as small GTP-dependent proteins and multiple proteins such as Rac and Rho, which are GTP-dependent regulatory proteins. The signal inducted by GPVI lead to the activation of phospholipase Cg2 (PLCg2). The activation of PLCg2 and the increase of intercellular Ca+2 stimulate the generation of the Ca+2 -sensor STIM1 and second messengers. In this way, Ca+2 ions are allowed to flow in the extracellular matrix and the circulating protein kinases are activated. GPIIb/IIIa (integrin aαIIbbβ3), the best known adhesion molecule in platelets, is commonly found in alpha granules, but they may be present on the surface of resting platelets in small quantities. However, with the activation of PLTs, the platelet surface is exposed to conformational changes leading to an increase in the number of the GPIIb/ IIIa receptors. As a result, tyrosine phosphorylation and phosphoinositol metabolism are activated and cell skeleton is reorganized in many proteins; and the formation of ADP and thromboxane A2 (TXA2) is triggered through degranulation. Eventually, adhesion scales up and adjacent platelets are summoned to the activation area resulting in the enlargement of the haemostatic plug. Additionally, the platelets rising to the high-affinity status from the low-affinity status binds not only to fibrinogens but also to matrix proteins involving ArgGly-Asp-Ser such as vWF. On the other hand, vWF binds to glycoprotein Ib/IX/V complex mediating the “outside-in” signaling. It also gives rise to conformational changes the PLT fibrinogen receptor glycoprotein IbIIa area and PLT activation occurs in the damaged domain. Ultimately, PLTs spread into the subendothelial matrix to form a solid adhesion, complete PLT aggregation and clot retraction. [3].
Integrin a2b1 strengthens and stabilizes the adhesion of platelets to collagen. Integrin proteins also ensure the PLTto-PLT binding, and at the same time, participate in contactdependent signaling of PLTs. Besides platelet integrin IIb3a, the surface receptor/ligand pairs such as semaphorin 4D, ephrin/ eph kinases and Gas6/TAM receptors and also the junctional adhesion molecules including platelet endothelial cell adhesion molecule (PECAM), junctional adhesion molecule-A (JAM-A) and endothelial cell-specific adhesion molecule (ESAM) play a role in this process. Ephrin B1-EphA4 enhances the activation of aIIbb3 and prevents platelet disaggregation. Semaphorin 4D/ plexin achieves both the contact-dependent signaling and the platelet-to-platelet interaction. A defect in these proteins can potentially hinder the formation of a solid thrombus. Reaction of Gas6 molecule, CD40L membrane protein, tetraspanins, ADP with platelet P2Y12 receptor induces integrin activation [4].
Thrombin, which is the most potent platelet antagonist, is found on the platelet cell surface and interacts with proteaseactivated receptors (PARs). In other words, thrombin activates platelets through serine protease - PAR1 and PAR4 receptors. PARs are G-protein coupled receptors, which provide the activation of PLTs through inositol phosphate metabolism and increased intracellular calcium. PAR-1 responds to low thrombin concentrations; activates myosin light chains with Rho-dependent phosphorylation and leads to alterations in the skeleton of the activated platelet. As a result, pseudopod generates to provide contact for collagen and other PLTs. Whereas, PAR-4 responds to higher thrombin concentrations. Platelet GPIb receptors contain a high-affinity binding site for thrombin and serve in thrombin signaling.
The increase of Ca+2 ions is necessary for granule release from platelet, integrin aIIbb3 activation, skeleton remodeling and externalization of platelet membrane phosphatidylserine. This externalization is a prerequisite for the procoagulant activities of the platelets [5]. Additionally, Ca+2 also participate in local thrombin formation by combining with coagulation factors.
Relation between Cellular Hemostasis and Free Radicals
Despite being indispensable for life, oxygen molecules form intermediates during metabolism which are highly reactive and known to be a resource for free radicals. Free radicals are reactive molecules that are generated during conversion of nutrients into energy by using oxygen. Reactive oxygen species (ROS) are generated by the superoxide anion from mitochondrial respiratory chain, hydrogen peroxide (H2O2) formed by phagocytic oxidases (PHOX) in the plasma membrane, and hypochlorous acid catalyzed by myeloperoxidase in active neutrophils. The control of redox hemostasis is the major determinant of cell fate. As there is a shift towards the pathological path from the physiological framework because of redox shifts in the small intracellular and extracellular environments, these molecules, known as reactive oxygen species or metabolites, damage cellular components such as lipids, proteins and DNA. Endothelial dysfunction caused by ROS underlies many diseases threatening people currently, such as atherosclerosis, hypertension (HT) and hyperlipidemia (HL). ROS also play a role in the activation of megakaryocytes. At high concentrations, ROS molecules cause leakage of intracellular content due to the damage of membrane. For instance, a H2O2 concentration >0.1 mmol/L has devastating effects. On the other side, non-mitochondrial production of ROS may also occur via potential ROS donors such as xanthine oxido-reductase, cyclooxygenase, uncoupled nitric oxide (NO) synthases, P450 mono-oxygenase, and lipoxygenase. Peroxynitrite targets cellular oxidation of carbon dioxide, hemoproteins and thiol as well as CO2 - dependent indirect oxidations. Peroxynitrite induces platelets by increasing thiol oxidization and intracellular Ca+2. In fact, the problem is that peroxynitrite causes more intense tyrosine phosphorylation than thrombin does. However, peroxynitrite at low concentrations (3-10 µm) inhibits aggregation through stimulation of ADP, thrombin, collagen and other PLT compounds. Furthermore, dual-acting peroxynitrite activates platelets in normal buffer, but vice versa in the presence of plasma.
Generation of ROS from eNOS
Endothelial nitric oxide synthase (eNOS) is a homodimeric enzyme with reductase and oxygenase domains. The dimer BH4 on the surface of eNOS is the binding site for tetrahydrobiopterin (BH4), and the substrate is L-arginine. NO is produced by the oxidation of arginine in a two-step reaction that generates L-citrulline and NO. The elevated peroxynitrite level resulting from the increase of oxidative stress leads to the formation of biologically inactive BH2 by the oxidation of BH4 which can no longer be used by the cell. As a result, uncoupled enzymatic status appears in which oxygen is converted into superoxide. Consequently, NO can be no longer synthesized.
Platelet ROS production
Besides the ROS generated by exogenous sources, platelets themselves also generate ROS. Platelets are the sources for ROS/RNS with their compounds containing superoxide anion and nitric oxide. Marcus AJ et al. [6] defined platelet-originated superoxide in 1977. In the following years, other ROS including O, OH, and HO were also discovered and their roles in PLT activation were revealed. Seno T et al. [7] indicated two subunits of NADPH oxidation in platelets, namely p22phox and p67phox, in 2011. Afterwards, the pathway that activates the thrombin receptor by inducing membrane translocation by platelet P67phox via phosphatidylinositol 3-Kinases (PI3- kinase) was introduced [8]. In this way, it was indicated that NADPH oxidase-dependent platelet releases O-2. NADPH oxidase in the platelets produces nanomolar quantities of isoforms localized in the membrane (gp91phox, p22phox) and in the cytoplasm (p47phox, gp67phox, and p40phox). Additionally, platelets contain ROS sources such as COX1, xanthine oxidase and eNOS.
The endothelial XO, COX, eNOS, NADPH oxidase, and CYP2C9 enzymes induce the production of oxygen radicals such as H2O2, O2 , and OH ions. ROS compounds not only activate the enzymatic systems in PLTs, but also contribute to ROS production process through thrombin and membrane depolarization induced by the activation of the NADPHoxidase subunits including NOX, p 91(NOX2) -22-67-47 phox.
Platelet NADPH-oxidase activation, which leads to plateletmediated LDL oxidation, plays a key role for the production of O2-. NADPH oxidase brings about reduced calcium mobilization, platelet activation and increased number of GPIIb/IIIa receptors [9]. Xanthine oxidase is another potential ROS source, but its role in platelet physiology is unclear. However, platelet activation has been inhibited by Allopurinol, a xanthine oxidase inhibitor. Isoprostane is a specific product of arachidonic acid oxidation and effective in thrombus growth as well as the late phase of PLT activation. 8-iso-PGF2 promotes the accumulation of PLTs within the vascular damage area.
Superoxide strengthens the recruitment of platelets to the growing thrombus. When platelets contact with the subcellular matrix proteins like collagen, the resting oval platelets begin to adhere to the endothelium. Activated bizarrely shaped PLTs release TX and ADP and attract other resting PLTs in the circulation to the same area. Stimulated PLTs initiate the release of ROS such as superoxide (O2-) and continue to grow by both inhibiting the thrombus formation and ADP breakdown and promoting NO destruction. Normally, NO prevents PLT adhesion by increasing the cGMP level in platelet. Platelet superoxide release can be hindered with GPIIb/IIIa inhibitors.
The Role of ROS in Platelet Signaling
ROS molecules directly activate platelets [10,11]. When platelets are activated, dense core granules start releasing ADP, serotonin, and Ca+2 while alpha granules release CD 40L, MMP 2/9, P selectin, RANTES, PDGF, TGF beta and PF4.
Platelet-originated O-2 is a physiological exterminator of platelet-derived NO, meaning inhibition of the anti-aggregant effect of NO. In other words, the higher the superoxide level is, the lower the NO level becomes which generates a tendency towards aggregation. For example, GPIIb/IIIa inhibitors obstruct the release of O-2 from PLT while strengthening NO release.
Adhesion of ROS platelets to vascular endothelium occurs with the destruction of the endothelial glycocalyx through the contribution of ADP resulting from the inactivation of endothelial ectonuclease. Moreover, ischemia-reperfusiondependent endothelial activation also contributes to that process. Thiol that is involved in the integrity and function of platelet affect the signaling of thiol redox switches, the oxidation-converting proteins.
With the stimulation of collagen, platelet can produce O-2 within 3-5 minutes, but the role of O-2 in the initial phase of aggregation is minimal. However, in the late phase of aggregation, the amount of O-2 increases with the elevation of ADP and integrates other PLTs in the process.
ROS influence the coagulation cascade and fibrinolysis through via their effects on several components of the cascade. Because of increased oxidative stress, platelets become more procoagulant and aggregant. Platelets bind extracellular macromolecular ligands such as fibrinogen and von Willebrand factor. Aggregated platelets have PS externalization, low calcium concentration, normal size, mitochondrial membrane potential, and cytoplasmic membrane integrity.
Platelet Dysfunction after Trauma
Platelets undertake a central role in cell-based hemostasis and play an indispensable role in maintaining hemostasis by creating 2/3 of the clot’s strength in trauma-mediated coagulopathy. The major causes of dysfunction include impairment in platelet adhesion, ADP response, PLT-to-PLT interaction, G protein signaling and local conditions. The PROPPR study reported that moderate or even mildly decreased platelet aggregation is strongly associated with mortality [12]. It was also indicated that platelet count on admission to hospital was inversely correlated with mortality even in the trauma patients in whom platelet function and coagulation parameters were normal. Therefore, the role of platelets in traumatic coagulopathy is obvious.
The small GTPase Rap1 plays a critical role in PLT adhesion [13]. In case of trauma, the antagonistic balance between GTPase Rap1, RASA3 and CalDAG-GEFI is significantly important for the modulation of PLT adhesions both in circulation and damaged area [14]. The RAP1 activator CalDAG-GEFI is very sensitive to small changes in cytoplasmic Ca+2 concentration and the activation response is the basis for the hemodynamic shear stress environment for an effective PLT adhesion. The RAP1 inhibitor RASA3 ensures that circulating PLTs remain stable against CalDAG-GEFI-dependent RAP1 activation. P2Y12 signaling inhibits RAP1 activation to form a stable haemostatic plaque. The increase of intracellular Ca+2 concentration triggers rapid activation of RAP1. Whereas, continuous RAP1 activation requires protein kinase C, P2Y12 and IP3 kinase signaling. RASA3 activity is down regulated in case of PLT activation which requires CalDAG-GEFIindependent, PKC/P2Y12-dependent signaling. RASA3 mutant platelets do not require P2Y12-mediated feedback, and their aggregation abilities are not affected by P2Y12 inhibitors.
Excessive release of ADP into the circulation as a result of tissue damage and hypo perfusion impairs the ADP response of platelets, and platelet abnormality may be the earliest indicator after injury.
In the cases such as trauma, inflammation and hypo perfusion, ROS is released by the cells such as platelets leukocytes, and endothelial cells. The release of ROS has a major impact on coagulation, and PAI-1, protein C and thrombomodulin are affected by oxidation. The formation of ROS and RNS during trauma causes direct endothelial damage and increases the concentration of potent PLT activators such as vWF and thrombin [15]. Kutcher et al. [16] employed thrombelastography (TEG) in their study and found a significant reduction in PLT aggregation response to endogenous agonists such as ADP in 45% of trauma patients. The trauma patients with impaired ADP pathway showed increased sensitivity to tPA and proteins released from parted platelets.
After ischemia-reperfusion (IR) injury, leukocytes and platelets accumulate around the postcapillary venule. The inter-endothelial junction expands and contraction occurs in cell skeleton. With the participation of the inflammatory response markers including IL2, IfN gamma, superoxide anion, hydrogen peroxide, TNF alpha, IL I, His, IL 6, and macrophage inflammatory protein into the process, the cell barriers collapse.
Platelet endothelial cell adhesion is provided directly by GPIb integrin (CD42) and indirectly by GPIIb/ IIIa integrin. During IR, these adhesion proteins located in PLT stimulate the expression of fibrinogen platelet in active endothelium, and moreover, the additional adhesion proteins including P and E selectin attract other potential destructive cells such as leukocyte to the relevant area. As a result, the damage expands; PLT and fibrinogen microtrombosis occurs in the endothelium; and endothelial damage deepens [17].
Besides increasing the cellular damage and promoting higher PLT activation, peroxynitrite, formed by the reaction of superoxide with NO, also enhances the endothelial damage and depends the severity of the damage by activating the signaling cascade of oxidative stress-induced apoptosis. Nicotinamideadenine dinucleotide phosphate (NADPH) oxidase (NOXs) is the major source of intracellular ROS [18]. Activation of PLT is triggered by ROS burst in ischemia-reperfusion, and moreover, the PLT eicosanoids, namely isoprostanes and TxA2, increase. Thrombin leads to the activation of Rho A/Rho Kinase (Rho/ ROCK) during IR [19]. Rho/ROCK inhibits the PI-3 kinase-Akt pathway which is responsible for cellular growth and apoptosis inhibition. Thrombin and Rho/ROCK-mediated inhibition of Akt (protein kinase B) impairs the phosphorylation of eNOS over Ser 1177 and leads to eNOS-mediated ROS production while reducing the NO presence. Rho/ROCK pathway reduces the expression and activation of eNOS.
It is known that coagulopathy is initially triggered by platelet hyperactivation in trauma, and subsequently, the stimulatory platelets become unresponsive. Platelets are exposed to tissue factor and PAF which brings them on the edge of extinction [20].
In packaged thrombocyte suspensions prepared for platelet transfusion, mitochondrial respiration worsens on day 2 of storage and ROS increases. Time-dependent platelet destruction results in platelet activation and dysfunction. With the reorganization of the cell structure, PLTs turns into dendritic from discoid. The GTPase Rap 1 content lessens GPIIb/IIIa activation and ADP response. Microvesicles disintegrate and become smaller [21]. Because of the altered expression of GpIb-IX-V receptors and metalloproteinases, GpIbα and GpV portions of the receptor vanishes resulting in the reduction of adhesiveness. Therefore, they are cleared from circulation early because of the failure of adhering to vWF.
Microparticles released from platelets
Microparticles (MPs) are small microvesicles with a diameter 0.1-1 μm and obtained from the cellular component of blood. MPs are defined as surface proteins and membrane lipids released from the originating cell in case of cell activation or cell death. Platelet-derived microparticles (PMPs), on the other hand, are the molecules, decreased levels of which may cause the reduction of clot strength and lead to death by increasing the need for transfusion even in the absence of a disorder related to aggregation and procoagulant responses [22]. PMPs have been indicated to give rise to MOF through proinflammatory effect in patients that died within 24 hours after trauma [23]. The generation of ROS and the increase of Ca+2 induce the externalization of phosphatidylserine (PS) through the activation of ATP-dependent flippases and nonspecific lipid transporters. PS is the phospholipid located in the monolayer of the resting PLTs. When the PLT is activated, PS leads to the shedding of plasma microparticles and transfers to external membrane. ROS/RNS may play a role in procoagulant MP formation by influencing Ca+2 hemostasis. Energy deficiency of platelet is associated with the increased shedding of thrombogenic MPs, and results from the reduction of enzymatic activities in mitochondrial electron transport chain. This situation will ultimately end in PLT apoptosis.
In brief, ROS exposure causes alterations in physiology and number of platelets. Mitochondrion plays a central role in these alterations and mediates changes in the cellular redox state by producing ROS/RNS. During trauma, the formation of ROS/RNS leads to direct endothelial damage and elevates the concentration of potent platelet activators such as thrombin. Under increased oxidative stress, platelets become more procoagulant and more aggregant. There is a need for further research on the antioxidants that can be used in therapeutic approaches and the potential of the currently used antiaggregant medicines for inhibiting oxidative stress.
- Krötz F, Sohn HY, Pohl U (2004) Reactive oxygen species players in the platelet game. Arterioscler Thromb Vasc Biol 24: 1988- 1996. [Ref.]
- Ferreira CN, Sousa M, Dusse LMS, Carvalho M (2010) A cell-based model of coagulation and its implications. Revista Brasileira de Hematologia e Hemoterapia 32: 416-421. [Ref.]
- Ferroni P, Vazzana N, Riondino S, Cuccurullo C, Guadagni F, et al. (2012) Platelet function in health and disease: from molecular mechanisms, redox considerations to novel therapeutic opportunities. Antioxid Redox Signal 17: 1447-1485. [Ref.]
- Stalker J (2014) Shaping the platelet response to vascular injury. Curr Opin Hematol 21:410-417. [Ref.]
- Versteeg HH, Heemskerk JW, Levi M, Reitsma PH (2013) New fundamentals in hemostasis. Physiol Rev 93: 327-358. [Ref.]
- Marcus AJ, Silk ST, Safier LB, Ullman HL (1977) Superoxide production and reducing activity in human platelets. J Clin Invest 59:149-158. [Ref.]
- Seno T, Inoue N, Gao D, Okuda M, Sumi Y, et al. (2001) Involvement of NADH/NADPH oxidase in human platelet ROS production. Thromb Res 103: 399-409. [Ref.]
- Chakrabarti S, Clutton P, Varghese S, Cox D, Mascelli MA, et al. (2004) Glycoprotein IIb/IIIa inhibition enhances platelet nitric oxide release. Thromb Res 113: 225-233. [Ref.]
- Violi F, Pignatelli P (2012) Platelet Oxidative Stress and Thrombosis. Thromb Res. 129: 378-381. [Ref.]
- Qiao J, Arthur JF, Gardiner EE, Andrews RK, Zeng L, et al. (2018) Regulation of platelet activation and thrombus formation by reactive oxygen species. Redox Biol 14: 126-130. [Ref.]
- Won KJ1, Lee HM, Lee CK, Lin HY, Na H, et al. (2011) Protein tyrosine phosphatase SHP-2 is positively involved in plateletderived growth factor-signaling in vascular neointima formation via the reactive oxygen species-related pathway. J Pharmacol Sci 115: 164-175. [Ref.]
- McCully BH, Connelly CR, Fair KA, Holcomb JB, Fox EE, et al. (2017) Onset of Coagulation Function Recovery Is Delayed in Severely Injured Trauma Patients with Venous Thromboembolism J Am Coll Surg 225: 42-51. [Ref.]
- Guidetti GF, Torti M (2012) The Small GTPase Rap1b: A Bidirectional Regulator of Platelet Adhesion Receptors. J Signal Transduct 2012: 412089. [Ref.]
- Stefanini L, Bergmeier W (2016) RAP1-GTPase signaling and platelet function. J Mol Med (Berl) 94: 13-19. [Ref.]
- Stefanini L, Bergmeier W (2016) RAP1-GTPase signaling and platelet function. J Mol Med (Berl) 94: 13-19. [Ref.]
- Kutcher ME, Redick BJ, McCreery RC, Crane IM, Greenberg MD, et al. (2012) Characterization of platelet dysfunction after trauma. J Trauma Acute Care Surg 73: 13-19. [Ref.]
- Dixon JT, Gozal E, Roberts AM (2012) Platelet-mediated vascular dysfunction during acute lung injury. Arch Physiol Biochem 118: 72-82. [Ref.]
- Sun HN, Kim SU, Lee MS, Kim SK, Kim JM, et al. (2008) Nicotinamide adenine dinucleotide phosphate (NADPH) oxidasedependent activation of phosphoinositide 3-kinase and p38 mitogen-activated protein kinase signal pathways is required for lipopolysaccharide-induced microglial phagocytosis. Biol Pharm Bull 31: 1711-1715. [Ref.]
- Yao L, Romero MJ, Toque HA, Yang G, Caldwell RB, et al. (2010) The role of RhoA/Rho kinase pathway in endothelial dysfunction. J Cardiovasc Dis Res 1: 165-170. [Ref.]
- Wohlauer MV, Moore EE, Thomas S, Sauaia A, Evans E, et al. (2012) Early Platelet Dysfunction: An Unrecognized Role in the Acute Coagulopathy of Trauma. J Am Coll Surg 214: 739-746. [Ref.]
- Melki I, Tessandier N, Zufferey A, Boilard E (2017) Platelet microvesicles in health and disease. Platelets 28: 214-221. [Ref.]
- Davenport RA, Brohi K (2016) Cause of trauma-induced coagulopathy. Curr Opin Anaesthesiol 29: 212-219. [Ref.]
- Stravitz RT, Ellerbe C, Durkalski V, Reuben A, Lisman T, et al. (2016) Thrombocytopenia Is Associated With Multi-organ System Failure in Patients With Acute Liver Failure. Clin Gastroenterol Hepatol 14: 613-620. [Ref.]
Download Provisional PDF Here
Article Type: REVIEW ARTICLE
Citation: Saracoglu A, Tetik S (2018) Reactive Oxygen Species and their Interaction with Platelets. J Med Chem Drug Des 1(1): dx.doi. org/10.16966/2578-9589.103
Copyright: © 2018 Saracoglu A, et al. This is an open-access article distributed under the terms of the Creative Commons Attribution License, which permits unrestricted use, distribution, and reproduction in any medium, provided the original author and source are credited.
Publication history:
SCI FORSCHEN JOURNALS
All Sci Forschen Journals are Open Access