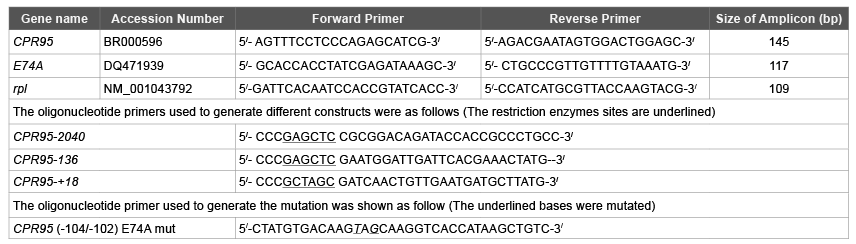
Table 1: List of Primers
Md. Saheb Ali1*† Birendra Mishra2 Mohammad Sahin Polan1† Osamu Ninagi3† Ahsanul Haque Swapon3 Masamitsu Yamaguchi4
1Agriculture Wing, Bangladesh Jute Research Institute, Manik Mia Ave, Dhaka, Bangladesh*Corresponding author: Md. Saheb Ali, Agriculture Wing, Bangladesh Jute Research Institute, Manik Mia Ave, Dhaka 1207, Bangladesh, Tel: +88-01714538138; E-mail: sahebbjri@yahoo.com
The present study was aimed to understand the regulatory mechanism of a cuticular protein gene coding for cuticle protein, CPR95 of Bombyx mori metamorphosis. Developmental profile of CPR95 resembled that of E74A. E74A and CPR95 transcripts induced by the 20E pulse treatment in vitro. Therefore, the results showed the relatedness of CPR95 and E74A. Site-directed mutagenesis of E74A binding site in the upstream of CPR95 and a reporter assay demonstrated the strong relatedness of CPR95 and E74A. Through genomic information and a transient reporter assay, we proved the regulating mechanism of CPR95 expression. The present findings showed that the temporal expression of CPR95 was regulated by ecdysone-responsive transcription factor E74A at the pre-pupal stage in the wing discs of Bombyx mori.
Temporal expression; Cuticular protein; Ecdysone pulse; Site-directed mutagenesis; Gene gun
Insect cuticle is a multi-layered structure with three functional regions: epicuticle, procuticle, and endocuticle, which differs in protein composition, structural features and physiological functions [1].The character of insect cuticle is determined by the construction of cuticular protein, and the various cuticle types show pronounced differences in mechanical properties and these differences are related to the properties of the individual proteins [2]. The character of insect cuticle differs in species and stages, and the difference is brought about by the combination of cuticular proteins. Cuticular proteins are characterized by repeated occurrence of a few small motifs consisting mainly of hydrophobic residues [2]. Thus, insects make up new cuticle at the each larval molting and different types of cuticle at the larval to pupal or pupal to adult transformation. Insect cuticle is mainly made up of chitin and cuticle proteins, and cuticular proteins determine the character of cuticle and bring about the variety of insect cuticle.
Ecdysone-responsive expression of cuticular proteins has been reported [3-12]. Most of their expressions were induced by an ecdysteroid pulse; the expression required the existence and removal of 20E [9,11,13]. This condition is similar to that of the stage around the ecdysis. Few examples were reported to be up-regulated by 20E [7,9,10]. They were induced by the addition of ecdysone invitro. Thus, cuticular protein genes, which have different developmental profiles, were induced by different types of ecdysone signal, and these things were described in a recent review [14].
Ecdysone-responsive transcription factors have been shown to determine the expression of cuticular protein genes [4]. βFTZ-F1 increased the promoter activity of the cuticular protein gene, BMWCP5, which was expressed around pupation when the ecdysteroid titer decreased after its peak [15,16]. BR-C Z2 activated the BmorCPG11 promoter independently from other ecdysone responsive transcription factors [6]. In addition to above transcription factors, EcR bound to the promoter region of the cuticular protein gene, BMWCP10, and activated its transcription [15]. BHR3 regulated BmorCPH5, and their transcription peak was observed earlier than that of BMWCP5 [4]. Thus, transcription factors bind to upstream of the target cuticular protein genes and bring about the stage-specific expression of cuticular protein genes in wing discs and epidermis. Therefore, cuticular protein genes are targets of ecdysoneresponsive transcription factors, as described above. These studies have been conducted by using a genomic database and hypothesized that the ecdysone-responsive transcription factor, E74A, regulates the expression of CPR95.
A hybrid strain of B. mori was reared at 25°C in a 12 h light: 12 h dark photoperiod. Larvae began wandering on six day of the fifth larval instar, pupation occurred 3 days thereafter, and adults enclosed 10 days after pupation. The periods (in days) corresponding to the developmental stages of the fourth to fifth larval ecdysis, wandering, pupation, and eclosion were designated as V0, W0, P0, and A0, respectively. The three days before pupation were designed as W1-W3. The W3 stage was divided into three different sub-stages, W3 early (W3E), W3 mid (W3M), and W3 late (W3L). The W3 sub-stages were determined on the time and visible shortening of the length of the leg [3].
Wing discs of larvae at W2 stages were prepared and cultured in vitro as previously described [4]. The culture was carried out at 25°C under sterile conditions according to a previous report [17].
To determine expression levels of cuticular protein gene and transcription factor, total RNA and cDNA synthesis were done according to a previous report [4]. Quantitative reverse transcription-PCR was performed using an ABI7500 real-time PCR machine (Applied Biosystems) using Fast Start Universal SYBR Green Master (Roche) according to the manufacturer’s protocol. The data were normalized in each sample using gene for the ribosomal protein S4. The oligonucleotide primer sets were designed using Primer3 software (http://frodo.wi.mit. edu/) as listed in Table 1.
Table 1: List of Primers
Upstream regions (-2040 to +18 and -136 to +18) of CPR95 were amplified by PCR according to a previous report [3]. The amplified DNA fragments were digested with SacI and NheI and then ligated into the SacI and NheI sites of the luciferase reporter plasmid pGL3- basic to generate the constructs. A Renilla luciferase reporter (PhRGhsp) driven by the Drosophila heat-shock protein70 promoter [18] was used as a normalization control [11]. Single mutant, (-104/-102) E74Amut for CPR95 was performed with the Quick-Change TM SiteDirected Mutagenesis Kit (Stratagene) using the CPR95-136 plasmid as the template. Twenty nanograms of plasmid DNA were amplified with Pfu DNA polymerase followed by digestion of the parental plasmid by DpnI. The mutated plasmid was introduced into XL1-Blue supercompetent cells. The mutagenic reactions were performed according to the manufacturer’s instructions. The introduction of each mutation was confirmed by sequencing. Oligonucleotide primers used to generate the constructs and mutation are shown in Table 1.
Transient expression of the reporter constructs in the wing discs was performed as previously described [4]. Following manufacturer’s instructions, 12.5 mg of gold particles (diameter: 1.0 µm) was coated with plasmid DNA (50 µg each of pGL3-derived vectors and 5 µg phRG-hsp). The reporter constructs were introduced into wing discs using a particle gun (Bio-rad). Bombardment proceeded under helium pressure of 150 psi (pounds per square inch). After bombardment, wing discs were cultured for 48 h at 25°C in Grace’s medium (Invitrogen) with or without 2 µg/ ml of 20E (Sigma). The culture method was described previously [17]. After 48 h of culture, wing discs were washed twice in PBS. The tissues were suspended in a 25 µl 1x reporter Passive Lysis Buffer (Promega) and frozen/thawed for 5 cycles in liquid nitrogen before the supernatant was equilibrated at 4°C for 1 h. The supernatant was collected by centrifugation at 12,000 g for 2 min at 4°C. The luciferase reporter assay was carried out using a Dual-Luciferase reporter assay system (Promega) in a luminometer (Perkin Elmer) according to the manufacturer’s protocol. The luciferase activity was normalized to the level of the Renilla luciferase activity. All experiments were performed at least five times. The results were expressed as the mean ± S. E. M., and significance was set at p <0.05.
Pieces of integument containing the head, thorax and abdomen (Figure 1) were dissected, fixed in Carnoy’s fixative for 2 h, and then dehydrated and embedded in paraffin. Seven-micrometer sections were de-waxed in xylene, rehydrated through an alcohol series, and then washed with tap water for 15 min. After washing, a Periodic acid Shiff (PAS) reaction [19] was performed to detect the polysaccharide derived from BM. The slides were then dipped in Lillie solution for 10 min (100 ml KIO4 + 0.4 ml of 60% HNO3 ), in Shiff ’s reagents for 30 min, and then in a H2 SO3 solution [5 ml of 1N HCl + 5 ml of 10% K2 S2 O5 + 100 ml H2 O] (3×1 min), and rinsed with tap water for 10 min. The sections were then stained with hematoxylin for 5 min, destained via immersion in acid alcohol (70% ethanol + 1 ml 1N HCl) for 1 min, and washed in running tap water for 15 min. After washing, they were dehydrated in a graded series of ethanol (70%, 90%, 95%, and absolute), cleared in xylene, and mounted using Canada balsam and a cover slip. The sections were visualized under a light microscope (Olympus BX50) and documented using DP-BSW software.
Figure 1: Bombyx larvae of W3 mid stage. A. An arrow indicates the dissected position for samples of thoracic epidermis. B. Arrows indicate the positions for samples of abdominal epidermis (A), wing disc (W) and head capsule (H).
A cuticular protein gene, CPR95 (Figure 2A), expressed in the fifth larval stage of Bombyx mori, which was similar to that of E74A. E74A is one of ecdysone-responsive transcription factor and showed expression peak at the W3L stage when ecdysteroid in the hemolymph declined at the pre-pupal period. Therefore, to understand the expression profile of E74A and CPR95, we analyzed the expression of E74A and CPR95.
Figure 2: Developmental profile of cuticular protein gene CPR95 (A) and ecdysone responsive transcription factor E74A (B). RNA was extracted from wing discs and reverse-transcribed to cDNA for use in Real-Time PCR. Values represent the mean ± S.E.M. of results from three independent experiments.
The expression of CPR95 increased rapidly after W3E stage and peaked at W3L stage, when the ecdysteroid titer in the hemolymph declining, then it rapidly decreased (Figure 2A). E74A transcripts increased rapidly after W3E stage and peaked at W3L stage then it rapidly decreased (Figure 2B), which is similar to the expression of CPR95. E74A and CPR95 transcripts rapidly decreased after W3L stage (Figure 2), when the hemolymph ecdysteroid titer decreased. Therefore the effect of ecdysone removal after treatment (ecdysone pulse) was examined. Transcripts of E74A increased after hormone removal (Figure 3A), which were not observed by the addition of cycloheximide. Transcripts of CPR95 also showed a similar increase after 20E removal (Figure 3B), and the addition of cycloheximide inhibited the transcription. The results suggest that the transcription of both genes was activated with the 20E removal. Both genes showed similar ecdysone pulse responsiveness; transcription was induced by the removal of 20E. From the similarity of expression profiles, it is suggested that CPR95 is induced by the ecdysone responsive transcription factor, E74A. Therefore we searched the upstream genomic sequences of CPR95 and found two putative E74A binding sites [4] upstream of CPR95 gene (Figure 4). Then, we applied reporter assay for the promoter analysis of CPR95 related with E74A.
Figure 3: Effect of ecdysone pulse treatment on E74A (A) and CPR95 (B). RNA was extracted from wing discs and reverse-transcribed to cDNA for use in Real-Time PCR. Wing discs of the W2 stage were incubated 12 h in a medium containing 2 µg/ml 20E and then transferred to a hormone-free medium with or without cycloheximide (50 µg/ml) for the indicated time. Values represent the mean ± S.E.M. of results from three independent experiments. Asterisks indicate p<0.05 significance by the student’s t-test.
Figure 4: Promoter activities of different lengths of the CPR95 5/ ‑flanking region. Luciferase reporter constructs having various lengths of the 5/ -flanking of CPR95 wild and mutated constructs were bombarded into wing discs of the W2 stage and cultured for 48 h with or without 2 µg/ml 20E. Numbers refer to the nucleotide position of the putative transcription start site. The luciferase activity was normalized to the Renilla luciferase activities. Luciferase assays were performed in five times, and results are reported as mean ± S.E.M. Firefly/Renilla ratios.
A plasmid containing a 2040 bp region upstream of CPR95 showed a higher promoter activity by the 20E pulse treatment (Figure 4). A deleted construct (CPR95-136) containing a putative E74A binding site showed a similar activity (Figure 4). Therefore, we conducted mutagenesis on this binding site using CPR95-136, resulted in the reduction of promoter activity (Figure 4). Luciferase activity of CPR95 promoter region in the media without 20E decreased to that cultured with 20E. This suggests mutation abolished the effect of 20E pulse that is suggested to be the effect of E74A. This result strongly suggests that the promoter activity of CPR95 was regulated by E74A.
Different expression patterns of cuticular protein genes in the wing discs of the fifth larval instar have reported and clarified the regulation of cuticular protein genes of Bombyx mori through the genomic database [4,11,15,20]. Here, we have clarified the related ecdysone-responsive transcription factors. The promoter region of the different cuticular protein genes was bound and activated by EcR and different ecdysoneresponsive transcription factors, βFTZ-F1, E74A, BR-C Z2, and BR-C Z4, resulting in their different expression patterns. These studies succeeded by using genomic information and a transient reporter assay by using wing discs.
In the present study, we found a cuticular protein gene, CPR95, which transcripts increased rapidly after the W3E stage and peaked at W3L, then rapidly decreased. Most cuticular protein genes showed expression peak at around the pupation [21], and transcription factors, βFTZ-F1, BR-C and E74A regulated the expression of these cuticular protein genes [4-6,15]. E74A was the only transcription factor that showed similar developmental profile to CPR95. CPR95 was induced by ecdysone pulse, and showed similar ecdysone responsiveness to E74A (Figure 3). E74A was induced by ecdysone pulse as previously reported in D. melanogaster [22], M. sexta [23] and Bombyx mori [4,24]. In the present study, we found the relatedness of CPR95 and E74A. CPR95 promoter was strongly regulated by the ecdysone pulse treatment, which disappeared by the mutagenesis of proximal putative E74A binding site (Figure 4). The result suggests the strong relatedness of E74A with CPR95. Together with the result of qRTPCR, we concluded that CPR95 transcription is regulated by E74A. It is suggested that the responsiveness to ecdysone determines the expression timing of transcription factors and their related cuticular protein genes, resulted in the difference of CPR95 and BmorCPH5expression [4]. Cuticular proteins containing R&R residue [25] are reported to bind with chitin [26,27] and would construct the procuticle. Although some of non R&R cuticular-proteins have the cuticle-binding capacity [28,29], most of them have been reported not to bind with chitin [30,31]. Most cuticular protein genes expressed in the wing discs are transcribed before pupation by the signal of ecdysone pulse, and most of them have R&R residue. CPR95 would construct procuticle, since it has R&R residue and is transcribed later than BmorCPH5 [4].
The expression of BR-Z2, βFTZ-F1 and E-74A was distinct in the epidermis of the head, thorax and abdomen respectively [5,6]. Histological photograph and the gene expression pattern demonstrated that different ecdysone-responsive transcription factors were expressed in the different region of the epidermis, which determined the cuticle protein genes expressed there (Figure 5). Thus, these ecdysone-responsive transcription factors are suggested to regulate their target genes, and the series of their expression would bring about insect metamorphosis.
Figure 5: Micro photographs of three different regions of epidermis. The integment of three regions shows different feature. Different types of epi-cuticle (ep) and pro-cuticle (pr) are observed. Nuclei (N) and basement membrane are in the base region.
The present findings suggest that cuticular protein genes are expressed in series according to their regulating transcription factors, resulting in a continuous series of cuticular protein production, which enable to construct the epi-, exo-, and endo-cuticle. These different types of cuticle proteins are combined and form the pupal cuticle, and the present findings suggest that ecdysone responsive transcription factors determine the space where cuticular protein genes are expressed. Genomic information and a transient reporter assay will further clarify the mechanism of insect cuticle construction.
Download Provisional PDF Here
Article Type: Research Article
Citation: Ali MS, Mishra B, Polan MS, Ninagi O, Swapon AH, et al. (2016) Regulation Studies of a Cuticle Protein Underlying Genomic Analysis. Int J Mol Genet Gene Ther 2(1): doi http://dx.doi.org/10.16966/2471-4968.104
Copyright: © 2016 Ali MS, et al. This is an open-access article distributed under the terms of the Creative Commons Attribution License, which permits unrestricted use, distribution, and reproduction in any medium, provided the original author and source are credited.
Publication history:
All Sci Forschen Journals are Open Access