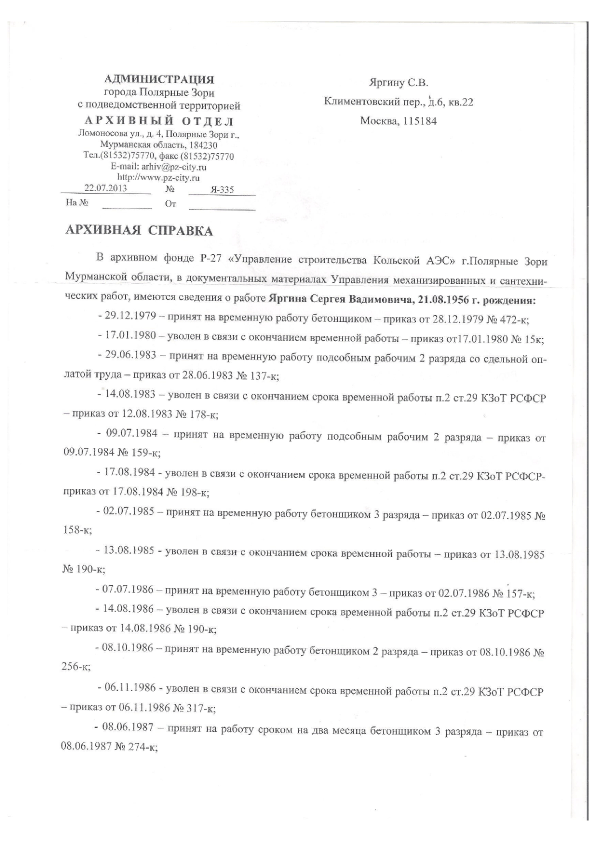
Figure 1: Certificate confirming repeated temporary employment with the Construction Management of the Kola Nuclear Power Plant. The dates can be seen. The factual working time was longer than indicated on the certificate.
Back to the Mechanisms of Cancer Incidence Increase after Chernobyl
Sergei V Jargin1* Andrei K Kaloshin2
1 Peoples’ Friendship University of Russia, Clementovski per 6-82, Moscow 115184, Russia*Corresponding author: Sergei V Jargin, Associate Professor, Peoples’ Friendship University of Russia, Clementovski per 6-82, Moscow 115184, Russia, Tel: +7 495 9516788; E-mail: sjargin@mail.ru
Article Type: Mini Review Article
Citation: Jargin SV, Kaloshin AK (2015) Back to the Mechanisms of Cancer Incidence Increase after Chernobyl. Int J Cancer Res Mol Mech 1(2): doihttp:// dx.doi.org/10.16966/2381-3318.105
Copyright: © 2015 Jargin SV, et al. This is an open-access article distributed under the terms of the Creative Commons Attribution License, which permits unrestricted use, distribution, and reproduction in any medium, provided the original author and source are credited.
Publication history:
After the Chernobyl accident appeared many publications exaggerating its medical consequences. Some of them are discussed in this minireview, which is a continuation of a preceding discussion. Among the motives for the overestimation were anti-nuclear resentments, widespread among the Green movement; however, their attitude has not been entirely wrong: nuclear facilities should have been prevented from spreading to overpopulated countries governed by unstable regimes and regions where conflicts and terrorism cannot be excluded. Nevertheless, we believe that certain Green activists have worked in the interests of the fossil fuel producers. The Chernobyl accident has been exploited to strangulate worldwide development of nuclear energy. Today, there are no alternatives to nuclear power: nonrenewable fossil fuels will probably become more expensive in the long run, contributing to the excessive population growth in the oil-producing countries and poverty in the rest of the world. Worldwide use of nuclear energy will become possible only after a concentration of authority within an efficient international executive based in the most developed countries. This will enable construction of nuclear power plants in optimally suitable places, considering all sociopolitical, geographic, geologic, and other preconditions, quality of working of local workforce, etc.
Chernobyl accident; Incidence; Thyroid cancer; Urinary bladder; Nuclear power plants
Dr. Janette D. Sherman wrote in her response [1] to my article [2]: “Until the independence of the WHO from the IAEA is assured, we can have little faith in their statements, whether it involves Chernobyl or Fukushima.” This mini-review continues the topic of independence with regard to the UNSCEAR: about 5 years ago during the author’s visit at the Medical Radiological Research Center in Obninsk, Russia, Prof. Victor K. Ivanov said in a conversation about his participation in the UNSCEAR Reports: “UNSCEAR, it is me!” In other words, political and economical interests sometimes overweigh scientific objectivity, which is perceivable from certain documents issued by highly esteemed international organizations. Chernobyl accident has been exploited to strangulate worldwide development of atomic industry [3]. Today, however, there are no alternatives to the nuclear power: in the long run, nonrenewable fossil fuels will probably become increasingly expensive, contributing to the uncontrolled population growth in the oil-producing countries and poverty in the rest of the world. It is also a question of independence from the fossil fuel producers, which is not irrelevant today, on the eve of the Independence Day.
Here follows an example. The registered incidence of Thyroid Cancer (TC) in children and adolescents in the former Soviet Union (SU) before the Chernobyl accident was lower than that in other developed countries [4]. This is not clearly perceptible from the literature because the increased TC incidence 4-5 years after the accident has been compared with that from the first years after the accident, when the registered incidence had already started to increase.
In Belarus during 1981-1985 the absolute number of TC diagnosed in children under 15 years was reported to be 3 and the average annual rate per million children - 0.3; in Ukraine, correspondingly, 25 and 0.5 [5]. For the northern regions of Ukraine, overlapping with the contaminated areas after the Chernobyl accident, the figures were 1.0 and 0.1 [5]. The above figures were reproduced in the Table 63 of the IARC Publication [6] with reference to [5]; but the rates were designated as “Rate/Million” which can be understood as a rate for the whole period (1981-85) and the entire population (e.g. 3 cases / 10 million inhabitants of Belarus=0.3 per 1 million); which, however, would be at variance with the original meaning in [5]. So it is written in [5,6]; but the incidence values seem to be inexact. According to the population pyramids for Belarus and Ukraine, children under 15 years around 1990 constituted a little more than 12 % of the entire population in both countries. Therefore, the annual rates per million would be up to: 3 cases / 5 years × 1.2 million children=0.5 for Belarus, and 25 cases / 5 years × 6 million=0.83 for Ukraine. For the northern Ukrainian regions the incidence rate would be around 0.17. In any case, the TC incidence rate in the former SU before the Chernobyl accident was considerably lower than the average for developed countries [4,7].
The incidence of pediatric TC for 1986 (the accident year) and subsequent years was presented in the Tables 56 and 57 of the Annex J to the UNSCEAR 2000 Report [8]. During 1986, 3 cases were registered in Belarus and 8 cases - in Ukraine. The incidence rate per 100,000 children under 15 years at diagnosis in 1986 was reported to be 0.2 (i.e. 2 per million) both for Belarus and Ukraine [8]. A calculation after the pattern from the preceding paragraph would result in 1.3 per million children for Ukraine.
The UNSCEAR 2008 Report compares the enhanced TC incidence rates 4 years after the accident and later not with the pre-accident level but with the years 1986-1990 (Annex D, pp. 60-61), when the incidence had increased up to 4.1 cases per million per year in people exposed at less than 10 years and 5.4-less than 18 years [9], which is considerably higher than the above-cited figures from [5,6]. The period 1986-1990 was chosen for comparison because “since 1986 and not earlier, specific data on thyroid cancer incidence have been specifically collected by local oncologists” (UNSCEAR Secretariat, personal communication of 22 October 2013). In the Russian Federation, TC was not registered separately till 1989 [10], when the large-scale screening had been started and the TC incidence began to increase dramatically. Considering the above, there was a pool of neglected TC in the population prior to the Chernobyl accident. The percentage of more advanced TC, sometimes interpreted as aggressive radiogenic cancers, was higher among the cases detected at an earlier date after the accident. This is apparently due to the detection by the screening of old neglected cancers, confirmed by the fact that the “first wave” TC after the Chernobyl accident were on average larger and less differentiated than those detected later [11]. The same must be true for renal cancer [12]. Further details are described in [4,13].
In the meantime, there continue to appear publications based on the Chernobyl material e.g. [14] commented in [15], as well as [16-18], where cause-effect relationship between radiation dose estimates and cancer risks is taken for granted. Without repeating the previously published arguments [4,7,12,13,15,19-24], the following should be stressed. Among the major causes of the cancer incidence increase after the Chernobyl accident were the screening with detection of neglected cancers accumulated in the population; classification of latent, dormant and borderline lesions as cancers; false-positivity; registration of non-exposed patients as Chernobyl victims; trimming of data. The incidence increase of TC after the accident can also be explained by its superficial location and availability for the screening, as well as by iodine deficiency in the contaminated territories accompanied by an increase in the prevalence of goiter [25,26], which was found by the screening and provided opportunities for over-diagnosis. The iodine supplementation was insufficient; although the iodine status of young people exposed to Chornobyl fallout in Belarus gradually improved along with improving iodine supplementation [26].
The motives for the exaggeration included writing of numerous theses, financing, etc. Moreover, Chernobyl accident was used as an instrument for strangulation of nuclear energy production [3] apparently with the goal of elevation of prices for fossil fuel. Mechanisms of false-positivity, pertaining e.g. to TC and urinary bladder lesions [4,13,15,19-22], were discussed previously. Among others, misinterpretation of nuclear pleomorphism as a malignancy criterion of thyroid nodules was not uncommon in the 1990s. In the urinary bladder, inflammatory reactive atypia was misinterpreted as dysplasia or carcinoma in situ [4,22]. On the basis of morphological descriptions and images from Russian-language literature on tumor pathology of that time, no reliable differential diagnosis could be made in some cases; illustrations are reproduced in [13,23,24].
In the author’s opinion, based also on the interviews with pathologists and other experts involved in the diagnostics of Chernobyl-related tumors, trimming of data contributed to the overestimation of Chernobyl consequences. A circumstantial evidence thereof is the large number of papers reporting obviously unrealistic data, partly referenced in [27] and commented in [24,28]. Special histopathological features of the Chernobyl-related pediatric TC should be commented. Almost all of them were of papillary type with a relatively high frequency of the solid and/or follicular pattern [29]. A reason thereof is obvious for a pathologist with a practical experience during the Soviet period. The diagnosis of follicular TC sometimes requires large number of thin histological sections from the capsular area of a nodule to search for capsular and vascular invasion [30,31], which was usually not done at that time because of technical reasons and insufficient awareness of the minimally invasive follicular carcinoma, not mentioned by Russian-language handbooks of that time. Therefore, if papillary TC tended to be over-diagnosed, follicular TC must have been under-diagnosed. Among others, false-positivity was caused by insufficient experience with pediatric TC and the celloidin embedding of specimens widely used during the 1990s [32]. In celloidin-embedded specimens, compared to conventional paraffin slides, cell nuclei appear somewhat “cleared” or ground-glass-like, which can be misinterpreted as a diagnostic criterion of papillary TC. Note that in the presence of the characteristic nuclear features a papillary TC can be diagnosed in the absence of papillary structures [30,31,33]. In my opinion, it is one of the reasons, why solid and follicular subtypes of papillary carcinoma were so frequent among Chernobyl-related TC cases [29]. Furthermore, it is known that advanced papillary TC often contain solid and/or follicular components. Accordingly, another cause of the relatively high frequency of the solid and follicular patterns in post-Chernobyl papillary TC must have been the fact that many cancers were relatively “old” i.e. at a later stage of tumor progression. Other morphological types of TC can be left out of discussion: medullary TC was very rare among the Chernobyl-related cases [34]; anaplastic TC, being more prevalent among the elders, has not been discussed in this context. Finally, regarding the absence of significant TC increase among children born after the Chernobyl accident, it should be commented that the data pertaining to them originated from a later period, when the quality of diagnostics improved, “radiation phobia” [35] subsided, and there were no motives to trim the data.
In the author reply [36] to my letter [37] it is stated in regard to cancer (in particular, lung cancer) incidence among Chernobyl cleanup workers (liquidators), coming from different regions of Russia, that “there is no correlation between difference in SIR estimates and life expectancy” in the corresponding regions. It would be important to know, in what way, reliably or not, the absence of the correlation was demonstrated, because the registered incidence of lung cancer should correlate with the coverage of the population by medical checkups using X-rays and the quality of the diagnostics [37], which in its turn would be logically associated with a higher life expectancy. It is furthermore stated in the author reply: “When internal comparison is made (radiation risk estimates), surveillance bias ‘was not operating,’ because all emergency workers (liquidators) of selected cohorts are covered by the similar level of health examination” [36]. However, the arguments from the letter [37] have remained unanswered: “It may be reasonably assumed that individuals knowing their doses were on average more motivated to undergo further medical examinations if a dose estimate had been relatively high; they were probably given on average more attention, and cared more about their health themselves. It is known that in the health care system of the former SU an extent of a medical checkup has sometimes depended on a patient’s initiative. ‘The dose dependent participation of self-reported pre-screening cases’ was mentioned in [38], which probably occurred in Chernobyl-related research of different kind [37]. In this connection, it is useful to recollect that, before claiming a cause-effect relationship, the question should be answered: “Is there any other way of explaining the set of facts before us, is there any answer equally, or more, likely than cause and effect?” [39]. Moreover, it should be questioned whether the facts have been trimmed or not, in particular, if the authors were noticed to use incorrect citation [40]. Further details on the claimed dose-effect correlations were mentioned by me [4,37].
Finally, the Linear Non-threshold Theory (LNT), pointed out in the author reply [36], should be commented. According to the LNT, the linear dose-effect correlation, proven to some extent for higher doses, can be extrapolated down to very low doses. The LNT is corroborated by the following arguments: The more particles hit a cell nucleus, the more DNA damage would result and the higher the risk of malignant transformation would be. Reducing the dose reduces the number of tracks and, correspondingly, the frequency of the effect [41,42]. This concept does not take into account that DNA damage and repair are normal processes in dynamic equilibrium. Accordingly, there must be an optimal level of impact i.e. of background radiation, as it is the case for light, UV, and many substances normally present in the environment. Any living organism would be best adapted by the natural selection to a level that occurs naturally, which is in accordance with experimental and epidemiological evidence in favor of radiation hormesis [43,44]. Natural selection is a slow process; adaptation to a changing environmental factor must therefore lag behind its current value, that is, correspond to some average from the past, especially for such an ancient mechanism as DNA repair. Natural background radiation has probably been decreasing during the time of life existence on the Earth, mainly due to the radionuclide decay on the surface and oxygen accumulation in the atmosphere, resulting in the ozone layer formation. Accordingly, living organisms must have been adapted to a higher background radiation level than that existing today; more details are in [20,45]. The form of the dose-response curve at the dose levels close to the natural radiation background can be further construed theoretically. There are different carcinogenic factors, both endo and exogenous. The lower would be added radioactivity due to contamination, the smaller would be the role of the contamination compared to the natural radioactive background, and the smaller would by the role of radiation as a whole compared to other carcinogenic factors and spontaneous carcinogenesis. Therefore, the dose-effect curve would deviate from linearity with a decreasing dose; the relationship can even become inverse in accordance with hormesis. A corresponding graph, plotted on the basis of experimental data, with a sagging of the doseeffect curve below the background cancer risk due to hormesis within the dose range 0.1-700 mGy, is depicted in the review [44]. Nevertheless, it is stated in the UNSCEAR Publication without references that the linear relationship “is consistent with most of the available mechanistic data” [46]. In conclusion, the LNT is not applicable to radiation doses comparable to those caused by the natural background. Dose-effect relationships after low-dose exposures should be studied in animal experiments with exactly known doses and dose rates, shielded from biases and vested interests.
It was mentioned in the abstract of this article that the quality of working is one of the factors determining where to construct nuclear power plants. It is known that insufficient respect for the formal requirements was among the causes of Chernobyl accident [35]. In the period 1979- 87, the author participated in construction of the Kola Nuclear Power Plant and the nearby town Polyarnye Zori (Polar Dawns) (Figures 1 and 2). In 1984 he participated in concrete works on the foundation for the Reactor No. 4, being a team leader for some period (Figure 3). Other workers: Timur Dzhanashwili (as far as we know, currently in the USA), Mikhail Selivanov; later joined Dmitrii Gotlib. The leader of the larger team, which included the above-named workers, was the engineer Andrei Kaloshin, who kindly agreed to be co-author of this paper. Concrete of special quality with coarse gravel was used for the foundation; it was poured down to the foundation pit with a rocky floor from mixer and other trucks. The workers leveled concrete with manual vibrators. Large amounts of concrete were often poured down at one moment, so that the quality of compaction was uneven. Occasionally the trucks poured into the foundation pit concrete of different quality if there was a leftover they could not dispose of elsewhere (Figure 4). Mikhail Selivanov had films and photographs of the works. It was supposed that he will print the photos for other workers, but since 1995 he refused to give them out and last time has been unavailable (Figure 4).
There are no conflicts of interest in connection with the above named article and the images.
Figure 1: Certificate confirming repeated temporary employment with the Construction Management of the Kola Nuclear Power Plant. The dates can be seen. The factual working time was longer than indicated on the certificate.
Figure 2: Certificate confirming repeated temporary employment with the Construction Management of the Kola Nuclear Power Plant. The dates can be seen. The factual working time was longer than indicated on the certificate.
Figure 3: A report to the Construction Management of the Kola Nuclear Power Plant on inadequate quality of concrete used for the foundation of the Reactor No. 4.
Figure 4: Report to the Police with a copy to the Rosatom Corporation asking to secure photographs and films in possession of Mikhail Selivanov. The photographs were regarded as common property of the workers, who were depicted on them.
Download Provisional pdf here
All Sci Forschen Journals are Open Access