Figure 1: Effects of dietary supplementation with medium chain glucomannan at 0.20% on intestinal microbiota of turkey hens at 30 days of age.
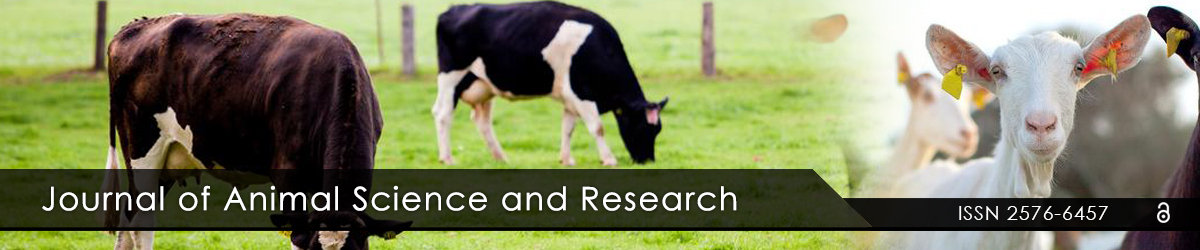
Full Text
Jean de Oliveira Guilherme Hosotani*
Cargill Research and Development Center Europe, Havenstraat, Vilvoorde, Belgium*Corresponding author: Guilherme Hosotani, Cargill Research and Development Center Europe, Havenstraat 84, 1800 Vilvoorde, Belgium; E-mail: guilherme_hosotani@cargill.com
Research into the use of prebiotics in poultry feed is widespread, including research into the application of fermentable oligosaccharides. Previous studies evaluating glucomannans demonstrated safety for broiler embryos, benefits on intestinal microbiota modulation, and inducing immune response. In this series of studies with broilers, layers, and turkeys, the effects on growth performance and egg production with feeding short, medium, and long chain glucomannans were evaluated. Glucomannans used in the current study were produced through acid polymerization of mannose monomers and tested in diets in a dose range from 0.02 to 2%. Current results show that chain length and dietary inclusion level of glucomannans provide species dependent effects. In broilers, the combination of studies indicated benefits on growth performance with short or long chain glucomannans at 0.02 and 0.20% in the diet, respectively. No major effects were observed in egg quality and production by layers fed either 0.02% short or medium chain glucomannans. In turkey hens, feeding diets with 0.20% medium chain glucomannan modulated intestinal microbiota, favouring the relative abundance of Lachnospiraceae and Bifidobacteria. Overall, in poultry species, incorporation of mannosepolymerized glucomannans with short, medium, and long chains at levels up to 0.20% resulted in improved growth performance in some of the studies depending on trial setup, challenge conditions and provided prebiotic effects.
Glucomannans; Mannan oligosaccharides; Poultry; Prebiotic; Nutrition
Finding alternatives to improve gut health and minimize foodborne pathogens in poultry production has been intensively investigated, mainly due to the limited or prohibited use of antibiotic growth promoters in animal feed.
Prebiotics are undigestible ingredients that are utilized by certain intestinal bacteria benefiting the host [1]. The use of prebiotics in animal feed is considered an effective method to increase beneficial bacteria in the microbiota of the gastrointestinal tract (GIT) of several animal species, including poultry [2,3] because prebiotics are utilized by the intestinal microbiota, they also have been reported to help in limiting pathogenic intestinal bacteria, which could be due to competition among bacterial strains, metabolites produced, or induced immune response by the host animal [4,5]. In addition, prebiotics can support microbiota maturation [4], indicating changes in the development of microbiota to a more complex and diverse composition, suggesting positive effects in health and growth performance in poultry [6]. Prebiotics also support animal growth by improving nutrient utilization, increasing enzyme activity and maintaining digestive function [7], controlling inflammation [8], and providing antioxidant activity [9].
Commercially available prebiotics typically include complex carbohydrates, yeast-derived mannan oligosaccharides (MOS), inulin, fructo-oligosaccharides (FOS), and wheat bran derived arabino-xylooligosaccharides (AXOS) [10-13]. Alternatively, glucomannans can be produced via proprietary technology of mannose polycondensation to create alternative oligosaccharide chain [4,14,15]. Glucomannans used in the current studies were produced using this technology for producing the short, medium, and long chain glucomannans, which differ in the level of degree of polymerization from low, medium, and high, respectively, with expected different effects on fermentation level, speed, gastrointestinal site, and microbiota modulation. According to each animal species digestive physiology characteristics (e.g., gastrointestinal length, digesta transit time, digestive secretions, microbiota, immune system), we hypothesized that specific glucomannans are indicated for each targeted animal species or mode of action.
Previous studies with these glucomannans demonstrated no adverse effects on embryonic growth and egg hatchability in broilers [4], and promoted intestinal microbiota modulation and induced immune response in vivo in broilers [15].
The objective of the current studies was to assess the effects of feeding glucomannans of short, medium, and long chain lengths at increasing inclusion levels on growth performance of broilers and turkeys, on intestinal microbiota of turkeys, and on egg production parameters in laying hens.
Glucomannan feed additives
Unlike MOS produced from yeast fermentation biomass by separation of cell wall fraction from Saccharomyces cerevisiae strains or produced from hemicellulose by enzymatic hydrolysis, glucomannans used in the current studies were produced through acid polymerization of mannose monomers from cereal grains in a microreactor, creating a material with greater purity regarding the oligosaccharide content and degree of polymerization or chain length. This technology allows the production of specific glucomannans for distinct applications in animal nutrition. In the current studies, glucomannans with low degree of polymerization were denominated short-chain glucomannans (SCG), glucomannans with medium degree of polymerization were the medium-chain glucomannans (MCG), and glucomannans with high degree of polymerization were the long-chain glucomannans (LCG).
General study description
Studies were performed either at global Cargill research facilities in different countries worldwide or at Cargill poultry integrators. Studies 1 through 6 were conducted with broilers and data were used for a meta-analysis of the effects of glucomannans in broilers, study 7 was performed with layers, and studies 8 and 9 with turkeys (Table 1). Broiler studies 1 and 2 were performed in the Netherlands, study 3 in France, studies 4 and 5 in the United States of America, and study 6 in Jordan. Layer study 7 was performed in India. Turkey hen studies 8 and 9 were performed in the United States of America. The studies met their respective local requirements for good animal husbandry and were supervised by licensed veterinary staff and/or trained animal scientists.
In all studies, basal feed was formulated to meet the nutritional requirements for the species and age of birds according to the NRC or to the breed catalogue. Diet compositions for the studies are shown in tables 2-4. Challenge conditions were applied in some studies and will be further mentioned (e.g., Salmonella inoculation), otherwise, no other intentional challenges were applied throughout the study (e.g., microbiological, feed quality, housing, and production conditions). In broiler studies, the length of the entire study was within the range of 20 to 35 d, however, the results shown were focused on the starter phase (0 to 20 ± 2 d of age) due to the expected effects of feeding glucomannans being greater at younger age and, consequently, the suggestion for adding glucomannans in the feed mainly during this period.
Study | Poultry | Treatment groups | Duration (days) | Replicates per treatment /Birds per replicate | Location |
1 | Broilers (Ross 308) | SCG-0.02% and 2.00% MCG-0.02% and 2.00% LCG-0.02% and 2.00% |
0-21 | 9/6 | Netherlands |
2 | Broilers (Ross 308) | SCG: 0.01%, 0.02%, 0.20%, 0.60%, 1.00% LCG: 0.01%, 0.02%, 0.20%, 0.60%, 1.00% | 0-28 | 12/6 | Netherlands |
3 | Broilers (Ross PM3) | SCG: 0.02% SCG: 0.02% SCG: 0.04% SCG: 0.04% SCG: 0.04% followed by SCG 0.02% |
0-18 0-35 0-18 0-35 0-18, 19-35 |
8/92 | France |
4 | Broilers (Ross 708) | SCG: 0.20% | 0-35 | 11/16 | United States |
5 | Broilers (Cobb x Cobb) |
SCG: 0.02% SCG: 0.02% |
0-22 0-35 |
16/16 | United States |
6 | Broilers (not specified) |
SCG: 0.02% |
0-28 No additive for days 29 - 35 |
9/50 | Jordan |
7 | Layers (BV 300) | SCG: 0.02% MCG: 0.02% |
90 | 7/10 | India |
8 | Turkey hens | MCG: 0.20% LCG: 0.20% |
7-48 | 15/23 | United States |
9 | Turkey hens | MCG: 0.20% | 0-46 | 15/21 | United States |
Table 1: Summary of treatment groups and duration for studies with short, medium, and long-chain glucomannans (SCG, MCG, LCG).
Study 1 | Study 2 (0-14d, 14-21d) |
Study 3 | Study 4 (0-8d, 8-22d) | Study 5 (0-8d, 8-15d, 15-22d) |
Study 6 (7-14d, 14-21d) |
|
Ingredients, % | ||||||
Corn | 17.83 | 20.92, 22.67 | 27.70 | 59.81, 60.23 | 37.36, 38.74, 38.81 | 32.20, 32.20 |
Wheat | 40.0 | 40.00 | 32.50 | - | 20.00 | 30.00 |
Rapeseed meal | _ | _ | 1.30 | - | - | - |
Soybean meal | 29.89 | 30.06, 27.74 | 33.10 | 33.45, 31.77 | 36.05, 35.87, 35.61 | 30.70 |
Animal fat | 2.61 | 1.65, 3.38 | 2.34, 3.67 | 2.68, 2.26, 3.03 | - | |
Soybean oil | 2.61 | 1.65, 1.11 | 1.00 | 2.80 | ||
Palm oil | - | - | 0.00 | - | - | - |
Limestone | - | 1.88, 1.53 | - | - | - | - |
Calcium carbonate | - | - | 0.00 | 1.56, 1.64 | 1.37, 1.21, 1.04 | 1.23 |
Monocalcium phosphate | 1.29 | 0.79, 0.59 | - | 1.34, 1.18 | 1.07, 0.71, 0.53 | 0.84 |
Dicalcium phosphate | - | - | 1.00 | 1.34, 1.18 | - | - |
Vitamin mineral premix | 1.00 | 1.00, 1.00 | 1.00 | 0.21, 0.21 | 0.21, 0.21, 0.19 | 0.58 |
Salt | 0.23 | 0.22, 0.22 | - | 0.40, 0.33 | 0.31, 0.33, 0.33 | 0.12 |
DL-Methionine | 0.20 | 0.19, 0.17 | 1.90* | 0.29, 0.28 | 0.27, 0.22, 0.19 | 0.42 |
L-Lysine | 0.12 | 0.14, 0.15 | 0.90* | 0.22, 0.21 | 0.24, 0.14, 0.06 | 0.37 |
Nutrients, % | ||||||
Crude protein | 20.97 | 20.60, 19.60 | 21.0 | 20.08, 19.32 | 22.12, 22.00, 21.77 | 20.7 |
Crude fat | 7.08 | 5.20, 6.40 | 3.10 | 4.39, 5.71 | 4.81, 4.43, 5.19 | 5.20 |
Crude fiber | 2.63 | 2.50, 2.50 | 3.20 | 2.03, 1.98 | 2.46, 2.48, 2.47 | 1.84 |
Dry matter | 85.71 | 86.30, 86.40 | 88.8 | |||
Calcium | 0.97 | 0.97, 0.80 | 0.76 | 1.07, 1.07 | 0.97, 0.85, 0.75 | 0.787, 0.787 |
Phosphorus | 0.68 | 0.55, 0.50 | 0.60 | 0.65, 0.60 | 0.45, 0.39, 0.36 | 0.527, 0.522 |
Table 2: General feed composition and nutrient composition data of diets in broiler studies.
*Feed additive with carrier
Study 7 (Pre-lay, Laying phase I)* | |
Ingredients, % | |
Corn | 44.96, 53.42 |
Rice | 10.00, 0.00 |
Soybean meal | 14.58, 17.27 |
Sunflower extract | 15.00, 15.00 |
Shell grit | 3.90, 6.04 |
Rice bran | 7.85, 3.77 |
Limestone | 1.90, 3.00 |
Dicalcium phosphate | 1.28,0.99 |
Vitamin mineral premix | 0.15, 0.15 |
Salt | 0.30, 0.30 |
DL-Methionine | 0.08, 0.08 |
Nutrients, % | |
Crude protein | 17.00; 17.50 |
Crude fiber | 7.16; 6.49 |
Calcium | 2.50; 3.60 |
Phosphorus | 0.40; 0.35 |
Table 3: General feed composition and nutrient composition data of diets
in layer studies.
*Pre-lay: 16-18 weeks; Laying phase I: 19-40 weeks
Study 8 | Study 9 (Control, MCG*) | |
Ingredients, % | ||
Corn | 38.22 | 38.22 |
Soybean meal | 43.20 | 43.20 |
Animal by-product meal | 7.30 | 7.30 |
Fat | 6.40 | 6.40 |
Calcium carbonate | 1.41 | 1.41 |
Monocalcium phosphate | 1.56 | 1.56 |
Vitamin mineral premix | 0.70 | 0.70 |
Salt | 0.21 | 0.21 |
DL-Methionine | 0.41 | 0.41 |
L-Lysine | 0.43 | 0.43 |
L-Threonine | 0.05 | 0.05 |
Nutrients, % | ||
Crude protein | 27.25 | 27.86, 27.13 |
Crude fat | 8.70 | 8.47, 8.90 |
Crude fiber | 2.14, 2.26 | |
Dry matter | 88.7 | 87.15, 87.64 |
Calcium | 1.42 | 1.69, 1.69 |
Phosphorus | 0.71 | 1.04, 0.99 |
Table 4: General feed composition and nutrient composition data of diets
in turkey studies.
*Medium-chain glucomannan treatment group.
Study 1-Broilers (The Netherlands)
A 21-d use rate-response feeding trial was performed to evaluate the effects of feeding glucomannan with different degrees of polymerization (SCG, MCG, and LCG) at 0.02% or 2.00% inclusion in the diet of Salmonella-challenged broiler chicks. A control group was included in which no glucomannans were included in the diet.
One-d-old, male broiler chicks were obtained from Morren BV Hatchery (Ross 308, n=54 per treatment group). Each treatment group had nine replicates with six birds per replicate. Birds were individually weighed and assigned to bioassay cages (50 × 50 cm; 12 birds per m2) with a raised wire floor covered by a metal plate and 2 cm of wood shavings, with each cage containing two nipple drinkers. For the first four days, feed was provided using a plastic feeder inside the cage. Subsequently, an adjustable feeder outside the cage was used. For the first 3 d, continuous artificial lighting was maintained for 23 h per d, which was reduced to 20 h per d between d 4 and 7, and 18 h per d for the rest of the trial. Temperature, relative humidity, and ventilation were computer controlled and varied as necessary to maintain optimal conditions. Environmental temperature was within the range from 34℃ at the start of the study until 24.6℃ at the end of the study, with 0.5℃ reduction per d.
A strain of nalidixic acid-resistant Salmonella enteritidis (SE) was grown overnight in brain heart infusion broth and diluted to 1.5 × 106 CFU/mL with buffered peptone water. At 6 d of age, feed was removed for 2 h, and all birds received 0.4 mL of the SE broth by gavage. Feed was then returned.
Body weight and feed consumption were measured at 0, 6, 17, and 20 d of age. The parameters average daily gain (ADG) and average daily feed intake (ADFI) were used to calculate feed conversion ratio (FCR). At 21 d of age, four birds per cage were randomly selected and euthanized via carbon dioxide asphyxiation, and ceca tissue, including contents, were collected for measurement of Salmonella levels.
Statistical analyses were performed for hypothesis testing and means separation. Effects were considered significant based on the probability of P<0.05. For comparison of the different treatments, all data were analyzed using a mixed-model approach using the PROC MIXED procedure in JMP (Version 9.2, 2008, SAS Institute Inc., Cary, NC). Models included the overall mean for the trait, the specific glucomannan and level tested, and the random effect of the block (location within experimental room), with pen as experimental unit. Salmonella CFU data were analyzed using a negative binomial distribution. The procedure was performed to accommodate the exponential scale of the CFU data. Samples with values below detection limit (<200) were set to 199 for analysis.
Study 2-Broilers (The Netherlands)
A 28-d use rate response feeding trial evaluated the effects of feeding two glucomannans (SCG and LCG), tested individually at five feed inclusion levels (0.01%, 0.02%, 0.20%, 0.60%, and 1.00%) under experimental non-challenged conditions. A control group was included in which no added glucomannans were included in the diet.
One-d-old male broiler chicks were obtained from Morren BV Hatchery (Ross 308; n=72 per treatment group). Each treatment group had six replicates with six birds per replicate. Birds were individually weighed and assigned to bioassay cages (50 × 50 cm; 12 birds per m2) with a raised wire floor covered by a metal plate and 2 cm of wood shavings, with each cage containing two nipple drinkers. For the first four days, feed was provided using a plastic feeder inside the cage. Subsequently, an adjustable feeder outside the cage was used. For the first 3 d, continuous artificial lighting was maintained for 23 h per d, which was reduced to 20 h per d between d 4 and 7, and 18 h per d for the rest of the trial. Temperature, relative humidity, and ventilation were computer controlled and varied as necessary to maintain optimal conditions. Environmental temperature was within the range from 34℃ at the start of the study until 22.1℃ at the end of the study (0.5℃ reduction per d for the first 13 d and by 0.4℃ per d for the remainder of the study).
Body weight and feed consumption were measured at 0, 7, 14, 21, and 28 d of age. Measured ADG and ADFI were used to calculate FCR. Average daily gain and total feed consumption were corrected for mortality and culling based on formulas provided by ID-TNO Animal Nutrition, Lelystad, the Netherlands. These formulas estimated feed intake of the dead or removed birds based on their final body weight, days in the trial, and the requirements for maintenance and weight gain [16].
At 28 d, three birds per cage were randomly selected, excluding obvious outliers, then weighed and euthanized using carbon dioxide. Cecum sample was collected and graded based on color (dark, light), consistency (liquid, liquid with stripes, solid, solid with stripes, empty), and presence of gas (none, some, foam, air).
Statistical analyses were performed for hypothesis testing and means separation. Effects were considered to be significant if the p-value was less than 0.05. For comparison of the different treatments, all data were analyzed using a mixed-model approach using the PROC MIXED procedure in JMP (Version 9.2, 2008, SAS Institute Inc., Cary, NC). Models included the overall mean for the trait, the specific glucomannan and level tested, and the random effect of the block. Mortality and ceca color score were analyzed as binomial variables, and ceca consistency and presence of gas as ordinal variables, both using PROC GLINMIX procedure in SAS.
Study 3-Broilers (France)
A 35-d feeding trial evaluated the effects of feeding 0.02% SCG from d 0 to18 or d 0 to 35, 0.04% SCG from d 0 to 18 d or d 0 to 35, or a combination of 0.04% SCG from 0 to 18 d followed by 0.02% SCG from 19 to 35 d of age of the birds. The study was performed under experimental and non-challenge conditions. A control group was included in which no glucomannans were added to the diet, and each treatment group had 8 replicates.
One-d-old Ross PM3 broiler chicks (n=736 per treatment group) were obtained from Boyé Hatchery. Each treatment group had 8 replicates with 92 birds per replicate (46 males and 46 females). Birds were individually weighed and assigned to floor pens (220 × 230 cm; 18 birds per m2) equipped with automatic water system with hanging water. Feed was provided ad libitum in crumble form from 0 to 18 d and pellets of 3.25mm from 19 to 35 d.
Continuous artificial lighting was initially provided for 23 h per d at d 1 of study and reduced daily until 18h per d at d 6, which was maintained until the end of the study. Temperature, relative humidity, and ventilation were computer-controlled and varied as necessary to maintain optimal conditions. The temperature was within the range from 33.5℃ at the start of the study until 18. 5℃ at the end of the study, with approximately 0.5℃ reduction per d.
Birds and feed consumption were measured at 0, 10, 18, and 35 d of age. Measured ADG and ADFI were used to calculate FCR. Mortality rate and water intake were also recorded.
Statistical analyses were performed for hypothesis testing and means separation. Effects were considered to be significant if the p-value was less than 0.05. For comparison of the different treatments, all data were analyzed using a mixed-model approach using the PROC MIXED procedure in JMP (Version 9.2, 2008, SAS Institute Inc., Cary, NC). Models included the overall mean for the trait, the specific glucomannan and level tested, and the random effect of the block.
Study 4-Broilers (USA)
A 35-d feeding trial evaluated the effects of feeding 0.20% SCG in the diets for broiler under simulated field conditions (reused litter material). A control group was included in which no added glucomannans were included in the diet.
One-d-old male broilers were obtained from Welp Hatchery (Ross 708; n=170 per treatment group). Each treatment group had 11 replicates with 16 birds per replicate. Birds were individually weighed and assigned to floor pens (89 × 117 cm; 15.37 birds per m2) equipped with automatic water with four nipples per pen. Feed was provided ad libitum in mash form in a feed tray for the first week and in a hanging tube feeder (one per pen) for the subsequent weeks. Continuous artificial lighting was maintained for 23 h per d throughout the experiment. Temperature, relative humidity, and ventilation were computer-controlled and varied as necessary to maintain optimal conditions. The temperature was within the range from 34.4℃ at the start of the study until 19.4℃ at the end of the study, with 0.5℃ reduction per d.
Average BW and feed consumption were measured at 8, 15, 22, 29, and 35 d of age. Based on measured BW gain and feed consumption, FCR was calculated for each period.
All data were analyzed using the PROC GLM procedure in SAS/ STAT software (Version 9.3, SAS Institute Inc., Cary, NC) as a complete randomized block design to compare treatment means.
Study 5-Broilers (USA)
A 35-d feeding trial evaluated the optimum feeding period for 0.02% SCG either from 0 to 22 d or 0 to 35 d. The study was performed under experimental and non-challenge conditions A control group was included in which no glucomannans were included in the diet.
One-d-old male broilers were obtained from Welp Hatchery (Cobb × Cobb; n=256 per treatment group). Each treatment had 16 replicates with 16 birds per replicate at the beginning of the study. Birds were individually weighed and assigned to floor pens (89 × 117 cm; 15.37 birds per m2) equipped with automatic water with four nipples per pen. Feed was provided ad libitum in pellet form in feed trays for the first week and in hanging tube feeders (one per pen) for subsequent weeks. Continuous artificial lighting was maintained for 23 h per day throughout the experiment. Temperature and ventilation were computer controlled and varied as necessary to maintain optimal conditions.
Average pen body weights and pen feed consumption were measured at 8, 15, 22, 29, and 35 d of age. Based on measured BW gain and feed consumption, FCR was calculated for each period.
All data were analyzed using the PROC MIXED procedure in SAS/ STAT software (Version 9.3, SAS Institute Inc., Cary, NC) as a complete randomized block design to compare treatment means. Least-square means analysis was used to separate treatment effects.
Study 6-Broilers (Jordan)
A 35-d feeding trial evaluated the effects of feeding 0.02% SCG in the diets for broilers. The study was performed under experimental and non-challenge conditions. A control group was included in which no glucomannans were included in the diet.
One-d-old broilers were obtained from a commercial hatchery (n=450 per treatment group). Each treatment group had 9 replicates with 50 birds per replicate. Birds were individually weighed and assigned to 5 m2 floor cages (10 birds per m2) equipped with automatic water through two bell drinkers per pen. Feed was provided ad libitum in a hanging tube feeder (one per pen) throughout the study. Feed additive (0.02% SCG) was only included in the feed from 7 to 28 d of age, with common feed from 1 to 6 d of age and from 29 to 35 d of age. Continuous artificial lighting was maintained for 23 h per d throughout the experiment. Temperature, relative humidity, and ventilation were computer-controlled and varied as necessary to maintain optimal conditions. The temperature was within the range from 32℃ at the start of the study until 20℃ at the end of the study, with 2℃ reduction per wk.
Average body weight and feed consumption were measured at 7, 14, 21, 28, and 35 d of age. Based on measured BW gain and feed consumption, FCR was calculated for each period.
The incidence of foot pad dermatitis was scored at 7, 14, 21, 28, and 35 d of age (0: normal; 1: mild lesion; 2: moderate lesion; 3: severe lesion). Wet litter was scored at d 14, 21, 28, and 35 (0: normal; 1: mild; 2: moderate; 3: severe).
All growth performance data were analyzed using PROC MIXED procedure in SAS/STAT software (Version 9.3, SAS Institute Inc., Cary, NC) as a complete randomized block design to compare treatment means. Scores for incidence of wet litter and foot pad dermatitis were analyzed as ordinal variables and using similar model as for growth performance data, with additional random effect of day.
Study 7-Layers (India)
A 12-wk feeding trial was performed to evaluate the effects of feeding SCG or MCG at 0.02% in the diet of layer hens. The study was performed under experimental and non-challenge conditions. A control group was included in which no glucomannans were included in the diet.
Eighteen wk-old laying hens were used at the start of the study (BV 300; n=70 per treatment group). Each treatment group had 7 replicates with 10 birds per replicate. Birds were allocated in individual cages equipped with nipple drinkers. Laying hens had ad libitum access to feed in mash form and water. Natural and artificial light was maintained for 16 h per d.
Feed intake, egg production, egg weight, albumin weight, yolk weight, shell weight, and shell thickness were measured weekly.
The study had a completely randomized design and data were analyzed using one-way ANOVA and Tukey’s test (JMP10, SAS software). Differences were considered significant if the p-value was less than 0.05.
Study 8-Turkey hens (USA)
A 41-d feeding trial was performed to evaluate the effects of feeding MCG or LCG at 0.20% in the diets for turkey poults. The study was performed under commercial conditions. A control group was included in which no glucomannans were included in the diet.
Seven d-old turkey hen poults were obtained from a commercial hatchery (n=345 per treatment group). Each treatment had 15 replicates with 23 birds per replicate. Birds were individually weighed and assigned to pens (1.22 m × 1.22 m; 0,065 birds per m2) with rice hulls and pine shavings as bedding material and equipped with hanging feeder and nipple drinkers. Feed was provided for ad libitum consumption in pellet form. Temperature, relative humidity, and ventilation were computer controlled and varied as necessary to maintain optimal conditions. The environmental temperature was within the range from 31℃ at the start of the study, with 0.1℃ reduction per d.
Average BW and feed consumption were measured at 7 and 48 d of age. The ADG and ADFI parameters were used to calculate FCR.
Microbial sample collection and analyses were performed according to the procedure previously described by Meijerink, et al. [4]. Digesta material was collected from two birds per pen at 30 and 48 d of age within all treatment groups using cloaca swabs. Following swab collection, samples were immediately stored at -80℃ prior to DNA extraction. The extracted DNA was labeled with a cy-5 fluorescentlabeled nucleotide to assess the relative abundance of a previously defined list of bacteria biomarkers by microarray analysis (Cargill Inc., proprietary). Comparison of the relative abundance of microbiota between treatment groups were determined based on the fluorescence intensity values from each probe.
Growth performance data were analyzed using R software, version 4.0.2, deploying nlme version 3.1-148 package for linear mixed effect models. Continuous normally distributed responses were modelled using a general lineal model including fixed effects of treatment groups and block as random effect. Least-square means analysis was used to separate treatment effects.
Microbiota dataset was submitted for data distribution analysis, followed by data standardization. The resulting dataset was subjected to ANOVA with array and block as random effects while bird age and treatment group were analyzed as fixed effects in a complete randomized block design. Comparisons were considered significant when FDR<0.05. Pairwise comparisons were used to produce volcano plots.
Study 9-Turkey hens (USA)
A 35-d feeding trial was performed to evaluate the effects of feeding MCG at 0.20% in the diets for turkey poults. The study was performed under commercial conditions. A control group was included in which no glucomannans were included in the diet.
Seven d-old hen poults were obtained from a commercial hatchery (n=315 per treatment group). Each treatment had 15 replicates with 21 birds per replicate. Birds were individually weighed and assigned to pens (1.22 m × 1.22 m; 0.065 birds per m2) with rice hulls and pine shavings as bedding material and equipped with hanging feeders and nipple drinkers. Feed was provided for ad libitum consumption in pellet form. Temperature, relative humidity, and ventilation were computer controlled and varied as necessary to maintain optimal conditions. Environmental temperature was within the range from 32℃ at the start of the study, with 0.1℃ reduction per d.
Average BW and feed consumption were measured at 7 and 42 d of age. The ADG and ADFI parameters were used to calculate FCR.
All data were analyzed using R software, version 4.0.2, deploying nlme version 3.1-148 package for linear mixed effect models. Continuous normally distributed responses were modelled using a general lineal model including fixed effects of treatment groups and block as random effect. Least-square means analysis was used to separate treatment effects.
General ingredient and nutrient composition of the diets from the studies on broilers, layers, and turkey hens are listed in tables 2-4, respectively. Summary growth performance data (BW, ADG, ADFI and FCR) of broilers during starter phase (0-20 ± 2 d) for each study are provided in table 5, and summary growth performance data of broilers for the entire study period are present in table 6. Summary of production performance data from layers and turkeys are listed in tables 9 and 10, res
Study 1 (0-20 days) | BW (g) | ADG (g) | ADFI (g) | FCR (g:g) |
Control | 924bcde | 43.9bcd | 55.6bcde | 1.266a |
SCG 0.02% | 997a | 47.5a | 60.6a | 1.275abc |
SCG 2.00% | 863fgh | 40.9ef | 53.1cdef | 1.300f |
MCG 0.02% | 939bcd | 44.7bcd | 56.9b | 1.273abc |
MCG 2.00% | 851gh | 40.3ef | 52.1ef | 1.294def |
LCG 0.02% | 946abcd | 45.1abc | 57.7ab | 1.281abcde |
LCG 2.00% | 888defg | 42.1de | 54.6bcde | 1.296ef |
SEM | 20.3 | 0.97 | 1.25 | 0.007 |
p-value | <0.0001 | <0.001 | <0.001 | <0.0001 |
Study 2 (0-21 days) | BW (g) | ADG (g) | ADFI (g) | FCR (g:g) |
SCG Control | 986ab | 45.1ab | 59.6ab | 1.32 |
SCG 0.01% | 978ab | 44.7ab | 59.2abc | 1.33 |
SCG 0.02% | 1,020a | 46.7a | 61.5a | 1.32 |
SCG 0.20% | 978ab | 44.7ab | 58.8abc | 1.32 |
SCG 0.60% | 950b | 43.3b | 57.3bc | 1.32 |
SCG 1.00% | 963ab | 44.0ab | 58.0abc | 1.32 |
LCG Control | 962ab | 43.9ab | 57.7bc | 1.31 |
LCG 0.01% | 979ab | 44.7ab | 58.3abc | 1.30 |
LCG 0.02% | 976ab | 44.6ab | 58.1abc | 1.30 |
LCG 0.20% | 991ab | 45.3ab | 59.1abc | 1.30 |
LCG 0.60% | 979ab | 44.7ab | 59.3abc | 1.33 |
LCG 1.00% | 930b | 42.4b | 55.7c | 1.31 |
SEM | 18.77 | 0.89 | 1.12 | 0.011 |
P-value | 0.003 | 0.003 | <0.001 | 0.283 |
Linear | 0.823 | 0.805 | 0.477 | 0.282 |
Quadratic | 0.004 | 0.004 | 0.0009 | 0.413 |
Study 3 (0-18 days) | BW (g) | ADG (g) | ADFI (g) | FCR (g:g) |
Control | 586 | 30.5 | 42.2 | 1.39 |
SCG 0.02% | 580 | 30.1 | 41.5 | 1.38 |
SCG 0.04% | 585 | 30.4 | 41.8 | 1.37 |
SEM | 27.3 | 1.49 | 1.35 | 0.04 |
P-value | 0.500 | 0.480 | 0.285 | 0.681 |
Study 4 (0-22 days) | BW (g) | ADG (g) | ADFI (g) | FCR (g:g) |
Control | 955 | 41.72 | 55.82b | 1.34a |
SCG 0.20% | 966 | 42.19 | 57.77a | 1.37b |
SEM | 8.47 | 0.38 | 0.52 | 0.01 |
P-value | 0.390 | 0.387 | 0.016 | 0.029 |
Study 5 (0-22 days) | BW (g) | ADG (g) | ADFI (g) | FCR (g:g) |
Control | 1,067 | 45.9 | 64.5 | 1.41 |
SCG 0.02%, 0-22d | 1,083 | 46.4 | 65.1 | 1.40 |
SEM | 7.5 | 0.35 | 0.53 | 0.002 |
P-value | 0.108 | 0.294 | 0.344 | 0.889 |
Study 6 (0-21 days) | BW (g) | ADG (g) | ADFI (g) | FCR (g:g) |
Control | 798 | 36.1 | 56.2a | 1.56b |
SCG 0.02% | 814 | 36.9 | 54.9b | 1.49a |
SEM | 8.36 | 0.39 | 0.171 | 0.015 |
P-value | 0.902 | 0.070 | <0.0001 | <0.001 |
Table 5: Summary of growth performance data for broiler studies 1 to 6 (starter phase; 20 ± 2 days of age).
a-f Means within a column without a common superscript differ (P<0.05) for each study.
Study 2 (0-28 days) | ADG (g) | ADFI (g) | FCR (g:g) |
SCG Control | 60.2ab | 84.1ab | 1.40 |
SCG 0.01% | 60.3ab | 83.6abc | 1.39 |
SCG 0.02% | 61.6a | 85.9a | 1.39 |
SCG 0.20% | 59.5ab | 82.3abc | 1.38 |
SCG 0.60% | 58.8ab | 81.0bc | 1.38 |
SCG 1.00% | 59.0ab | 81.7abc | 1.39 |
LCG Control | 58.9ab | 81.8abc | 1.39 |
LCG 0.01% | 59.3ab | 81.7abc | 1.38 |
LCG 0.02% | 59.6ab | 81.9abc | 1.38 |
LCG 0.20% | 59.2ab | 81.8abc | 1.38 |
LCG 0.60% | 60.1ab | 83.0abc | 1.38 |
LCG 1.00% | 58.1b | 79.6c | 1.37 |
SEM | 0.87 | 1.26 | 0.01 |
p-value | 0.016 | 0.0008 | 0.2055 |
Linear | 0.178 | 0.161 | 0.825 |
Quadratic | 0.019 | 0.003 | 0.077 |
Study 3 (0-35 days) | ADG (g) | ADFI (g) | FCR (g:g) |
Control | 48.9 | 80.6 | 1.65 |
SCG 0.02%, 0-35d | 47.1 | 77.2 | 1.67 |
SCG 0.02%, 0-18d | 47.1 | 78.2 | 1.66 |
SCG 0.04%, 0-18d and SCG 0.02%, 19-35d | 47.7 | 79.0 | 1.66 |
SCG 0.04%, 0-18d | 46.0 | 78.3 | 1.68 |
SCG 0.04%, 0-35d | 45.7 | 76.4 | 1.67 |
SEM | 2.17 | 2.97 | 0.02 |
p-value | 0.074 | 0.130 | 0.054 |
Study 4 (0-35 days) | ADG (g) | ADFI (g) | FCR (g:g) |
Control | 62.6a | 86.4 | 1.38a |
SCG 0.20% | 53.6b | 84.9 | 1.58b |
SEM | 0.713 | 0.784 | 0.005 |
p-value | <0.01 | 0.154 | <0.01 |
Study 5 (0-35 days) | ADG (g) | ADFI (g) | FCR (g:g) |
Control | 62.9 | 95.1 | 1.52 |
SCG 0.02%, 0-22d | 63.2 | 95.2 | 1.51 |
SCG 0.02%, 0-35d | 62.7 | 95.5 | 1.52 |
SEM | 0.70 | 1.06 | 0.005 |
p-value | 0.889 | 0.884 | 0.522 |
Study 6 (0-35 days) | ADG (g) | ADFI (g) | FCR (g:g) |
Control | 51.8b | 92.0a | 1.78b |
SCG 0.02% | 54.8a | 90.7b | 1.66a |
SEM | 0.425 | 0.050 | 0.0123 |
p-value | <0.0001 | <0.0001 | <0.0001 |
Table 6: Summary of growth performance data for broiler studies 2 to 6
(starter and grower phase; up to 28 or 35 days of age).
a-fMeans within a column without a common superscript differ (P<0.05) for each study.
Study 1-Broilers
The response of birds to the different glucomannans was mostly use rate dependent. Compared to the control group, feeding SCG at 0.02% for 20 d increased BW (997g vs. 924g; P<0.0001), ADG (47.5 g vs. 43.9 g; P<0.0001), ADFI (60.6 g vs. 55.6 g; P<0.0001), and FCR (1.28 g:g vs. 1.27 g:g; P<0.0001). Feeding MCG and LCG at 0.02% for 20 d did not affect BW, ADG, ADFI, and FCR compared to control group (P>0.05) to the same extend as SCG at 0.02%, with only numerical improvement on BW, ADG, and ADFI. Feeding SCG or MCG at 2.00% resulted in reduced BW (P<0.0001) and ADG (P<0.001), increased FCR (P<0.0001), and similar ADFI to control group (P>0.05), while feeding LCG at 2.00% resulted in increased FCR compared to control group (P<0.0001).
Salmonella counts were not different from the control for all inclusion levels of SCG, MCG, and LCG (data not shown). Average mortality and culling rate combined were at 2.1% with no differences among treatments in this study (P=0.998), which is low compared to the normal level of incidence in this research facility at 4.4%.
Study 2-Broilers
Feeding broilers with SCG or LCG for 21 d resulted in a quadratic response on growth performance on BW (P=0.004), ADG (P=0.019) and ADFI (P=0.003), with positive response for SCG included up to 0.02% and LCG up to 0.20% in the diet. Birds fed with SCG at 0.02% had BW of 1,020g compared to control group at 986g. A similar trend was observed for ADG (46.7 g vs. 45.1 g) and ADFI (61.5 g vs. 59.6 g), while FCR were similar (1.32 g:g vs. 1.32 g:g). Birds fed with LCG at 0.20% had BW of 991g compared to control group at 962g. A similar trend was observed for ADG (45.3 g vs. 43.9 g) and ADFI (59.1 g vs. 57.7 g), resulting in FCR at 1.30 g:g vs. 1.31 g:g for control group.
No differences were observed with feeding SCG or MCG at levels up to 1% of the diet on the probability of lighter ceca content (P=0.392) or the probability of less gas score in ceca (P=0.730). However, there was a quadratic response for the probability of softer consistency ceca score in percentage (P<0.05), with a reduction in the probability when both SCG and LCG were fed at levels up to 0.20% (Table 7).
Treatment | Probability of lighter ceca score (%) | Probability of softer consistency ceca score (%) | Probability of less gas score (%) |
SCG 0.00% | 30.3 | 72.6 | 71.3 |
SCG 0.01% | 44.3 | 84.3 | 82.4 |
SCG 0.02% | 58.5 | 82.2 | 66.6 |
SCG 0.20% | 44.3 | 81.4 | 74.0 |
SCG 0.60% | 55.7 | 83.9 | 72.7 |
SCG 1.00% | 67.0 | 88.7 | 82.4 |
MCG 0.00% | 33.1 | 79.8 | 68.3 |
MCG 0.01% | 58.5 | 85.4 | 72.0 |
MCG 0.02% | 44.3 | 84.6 | 69.5 |
MCG 0.20% | 55.7 | 83.3 | 61.0 |
MCG 0.60% | 67.0 | 93.3 | 71.7 |
MCG 1.00% | 55.7 | 83.3 | 72.1 |
P-value | 0.392 | 0.460 | 0.730 |
Contrasts | |||
SCG x MCG | 0.632 | 0.245 | 0.089 |
Linear | >0.05 | >0.05 | >0.05 |
Quadratic | >0.05 | <0.05 | >0.05 |
Table 7: Effects of increasing levels of glucomannan supplementation on visual cecum content score in broilers at 28 d of age (Study 2).
The combined mortality and culling rate in this study was at 4.5%, similar to the average value from previous studies in this research facility at 4.4%. There were no differences among treatments (P=0.523).
Study 3-Broilers
Overall, feeding broilers with SCG at 0.02% or 0.04% for 18 d did not result in differences in BW, ADG, ADFI, and FCR compared to control group (P>0.05). In this study, it was observed that the performance parameters, especially ADG and ADFI, were lower by 18% than typical performance in studies at this research facility due to poor quality chicks in comparison with previous studies at the same research facility. Despite the overall worse growth performance, the average mortality rate among treatment groups in this study was at 1.7%, which was considered low in this research facility. There were no differences among treatments (P>0.05).
Study 4-Broilers
Overall, feeding broiler chicks SCG at 0.20% during the first 22 d resulted in increased ADFI compared to control group (57.8 g vs 55.8 g; P=0.016), numerically increased ADG (42.2 g vs. 41.7 g; P=0.387) or BW (1,083 g vs. 1,067 g; P=0.108), and increased FCR (1.37 g:g vs. 1.34 g:g; P=0.029).
Although in this study an intentional stress factor was applied by reusing the litter from previous production cycle to increase the pressure on the growth performance, the average mortality rate among treatment groups was at 5.97%, similar among treatment groups (P=0.8032).
Study 5-Broilers
In this study there was no significant difference in broiler performance parameters (BW, ADG, ADFI, FCR) with birds fed SCG at 0.02% for 22 d compared to control group (P>0.05). However, feeding birds with SCG at 0.02% resulted in numerical improvement compared to control group in BW (1,083 g vs. 1,067 g; P=0.108), ADG (46.4 g vs. 45.9 g; P=0.294), ADFI (65.1 g vs. 64.5 g; P=0.344), and FCR (1.40 g:g vs. 1.41 g:g; P=0.889).
Study 6-Broilers
Birds fed SCG at 0.02% for 21 d had reduced ADFI compared to control group (54.9 g vs. 56.2 g; P<0.0001), numerically increased ADG (36.9 g vs. 36.1 g; P=0.070) and BW (814 g vs. 798 g; P=0.902), therefore, improved FCR (1.49 g:g vs. 1.56 g:g; P<0.001). These results are not entirely similar to results of previous studies showing increased feed intake with feeding SCG, however, with reduced feed intake and numerically greater ADG and BW, the feed efficiency was improved.
Overall, during the entire study, the probability of a higher foot pad dermatitis score was lower, at 0.059%, in broilers fed diets with 0.02% SCG compared to control group 0.198% (P<0.001; Table 8).
Treatment | Probability of higher litter score1 (%) | Probability of a higher foot pad dermatitis score2 (%) |
Control | 0.297 | 0.198 |
SCG 0.02% | 0.136 | 0.059 |
SEM | 0.070 | 0.045 |
P-value | >0.05 | <0.001 |
Table 8: Effects of feeding glucomannans on probability of higher scores
of wet litter and footpad dermatitis in broilers (Study 6).
1 Litter score: 0: normal; 1: mild; 2: moderate; 3: severe
2 Foot pad dermatitis score: 0: normal; 1: mild lesion; 2: moderate lesion; 3: severe lesion
Implications for Broilers
Results from study 1 demonstrated that in broilers challenged with Salmonella, the chain length of the glucomannans had different effects on growth performance, with SCG at 0.02% inclusion level being more effective on growth performance, increasing ADG by 8.2% compared to control group (47.5 g vs. 43.9 g per d, respectively), mainly due to increased feed intake (60.6 vs. 55.6 g per d, respectively). Therefore, based on the difference in the composition (degree of polymerization), each type of glucomannan is expected to behave differently within the gastrointestinal tract of animals and, therefore, may result in distinct modes of action, either on promoting increased feed intake or prebiotic effects. In addition, the inclusion level in the diet was also important and more effective at lower inclusion rate (0.02% of the diet), demonstrating that the functionality of the glucomannans is dependent on inclusion rate.
Results from study 2 were in agreement with study 1 (under Salmonella challenge conditions) and suggest that induced challenge conditions or under commercial setup conditions, the beneficial effects of glucomannans may be increased. The growth performance results during the starter phase in this study show that under non-challenge conditions, the optimal level of SCG is 0.02% and LCG is up to 0.20%, mainly driven by increased feed consumption. Quadratic response with reduction in the probability of softer consistency ceca score with SCG and LCG at levels up to 0.20% indicate an effect on nutrient digestibility, intestinal microbial fermentation, or a combination of these effects.
Results from study 4 agreed with studies 1 and 2, suggesting that SCG at 0.20% can promote improved growth performance parameters by inducing increased feed intake.
Although only numerically, results from study 5 also suggest that growth performance may be improved with feeding SCG at 0.02% during the first 20 ± 2 d of age.
In study 6, the observed positive effect on FCR might be related to the potential benefits of feeding SCG in a condition similar to commercial setup compared to ideal conditions of most research facilities from previous trials. Results also suggest that feeding SCG at 0.02% may improve nutrient digestibility, intestinal microbiota, intestinal health, immune response, and therefore, supporting health of birds, resulting in lower moisture in excreta or lower diarrhea incidence, leading to better litter quality and lower incidence of foot pad dermatitis.
Currently, the market of MOS for animal nutrition are mainly derived from yeast products from Saccharomyces cerevisiae and comprised of cell wall components, mostly of α-MOS from hydrolysis of α-1, 6-mannan from yeast cell walls [17]. Originally, prior to the process of yeast cell lysis and cell wall extraction, the main cell wall components are β1,3- and β1,6-glucans, polymers of mannose (mannans as mannoproteins) and chitin at approximately 60, 40, and 2%, respectively, with differences depending on yeast development, processing methods and conditions, and purity of product [18- 20]. Yeast cell walls should contain components with degree of polymerization at 1,500 for β1,3-glucans and 140 for β1,6-glucans [20]. Glucomannans used in the current studies contain greater concentration of mannans than yeast material sources due to starting material being mannose as opposed to yeast biomass or extracted yeast cell wall. Similar benefits have been demonstrated for mannan enriched products [21]. In addition, the technology used for mannose polycondensation allows for the design of glucomannans rich in specific degree of polymerization for different applications.
Meijerink, et al. [4] performed a series of in vitro, in ovo, and in vivo studies with selected chemicals and plant extracts to examine the effects on the intestinal microbiota and the immune system in broilers. Feed materials that potentially help activate natural killer cells and macrophages in vitro progressed to an in ovo study, including similar LCG investigated in the current studies. The LCG, at all levels (0.02%, 0.20% and 2.00% in saline solution) did not adversely affect embryonic growth and hatchability of the eggs [22]. Feed supplementation for broilers with LCG at 0.20% for 21 d did not adversely alter growth performance parameters and enhanced some immune function parameters (NK cell activation) at 7 d of age and resulted in an increase in Lactobacillus species in the intestinal microbiota.
Meijerink, et al. [15] also investigated the effects of LCG in broiler diets following Salmonella enterica infection. Among the findings, feeding LCG at 0.20% induced immune response (increased number and activation of intraepithelial NK cells, cytotoxic CD8+ T cell numbers, higher Salmonella enterica specific antibody response), modulated intestinal microbiota (increased relative abundance of lactic acid producing bacteria in microbiota of the ileum and caeca), and reduced Salmonella enterica prevalence in broilers.
Previous studies with mannan oligosaccharides from Saccharomyces cerevisiae also have shown modulation of intestinal microbiota in broilers, with increase in lactic acid bacteria [4,23], reduction in E. coli [23,24], and reduction in Salmonella [15]. E. coli and Salmonella, gram-negative bacteria colonize intestinal mucosa via attachment with type-1 fimbrial adhesive, which mediates binding to N-linked oligosaccharides [25]. Mannan oligosaccharides can reduce bacteria attachment to the intestinal mucosa through competition for receptors in intestinal membrane, favoring bacteria to move along the intestinal tract [26]. In addition, mannan oligosaccharides may increase the number of intestinal goblet cells, responsible for secreting mucins that can also bind to bacteria, reducing their colonization [23,27].
Numerous feeding studies in the literature support the functionality and safety of MOS different than the glucomannans tested in the current studies. Baurhoo, et al. [27] found that inclusion of MOS in the broiler diet (0.20% to 21 d and 0.10% to 22-42 d) led to BW and feed intake similar to controls up to 35 d but decreased significantly at 42 d, while feed conversion during the study was not adversely affected. In addition, birds fed MOS had increased beneficial bacteria, Lactobacilli and Bifidobacteria, in the GI tract compared to control. Corrigan, et al. [28] fed broilers 0.10% or 0.20% MOS in the diet for 42 d and found no adverse effects on performance (BW, feed consumption, and FCR). Feeding MOS was found to have significantly changed the cecal microbiota for the MOS treatment groups compared to control group. Kim, et al. [29] included fructo-oligosaccharide (FOS; 0.25% or 0.50%) or MOS (0.025% or 0.05%) in broiler diets for four weeks and found no significant differences in feed intake, FCR, and mortality compared to control. Favorable alterations in the intestinal microbiota were also observed (decrease in Clostridium perfringens and Escherichia coli and increases in Lactobacilli with 0.25% FOS and 0.05% MOS). Ao and Choct [30] fed diets supplemented with either MOS or FOS (0.10% starter diet and 0.05% in grower-finisher diet) and observed increased BW and FCR compared to control group after 21 and 35 d. Rehman, et al. [31] fed broilers 0.10% or 0.15% MOS in the diet from 0 to 35 d of age and found no change in feed intake, increased BW gain, and lowered FCR compared to control group over the entire period.
Data from the six broiler studies during starter phase (20 ± 2 d) suggest that there is specificity of glucomannan chain length, and inclusion levels are specific for improving broiler growth. In five studies in which broilers were fed with SCG at 0.02% for 20 ± 2 d, the average BW, ADG, ADFI, and FCR were 899 g, 41.5 g, 56.7 g, and 1.37 g:g, respectively, while the average BW, ADG, ADFI, and FCR for control group were 872 g, 40.3 g, 55.6 g, and 1.39 g:g, respectively. Therefore, SCG at 0.02% has been shown to improve BW, ADG, ADFI, and FCR by 2.8%, 2.7%, 1.8%, and 1.04%, respectively compared to control group (studies 1, 2, 3, 5, and 6). In comparison, feeding MCG at 0.02% resulted in improved BW by 1.6%, ADG by 1.8%, ADFI by 2.3%, and impaired FCR by 0.55% compared to control group (study 1), while LCG at 0.20% improved BW by 3.0%, ADG by 3.2%, ADFI by 2.4% and FCR by 0.76% (study 2).
Based on the current studies on broilers, it can be inferred that glucomannans may improve growth of broilers by inducing increased feed intake, mainly glucomannans of short chain length. Increased feed intake may be driven indirectly by immune system activation, improvement in integrity of intestinal tract, or changes in intestinal microbiota as these factors play a central role in maintaining feed intake under challenge conditions from nutrition or environment [32]. Glucomannans of long chain length can provide prebiotic effects as observed in previous studies with broilers or turkeys [4,14,15], with glucomannans of short and medium chain length showing prebiotic effects to a lesser extent.
In addition, litter quality may be improved with feeding SCG at the suggested inclusion level of 0.02% in the diet (Study 6), being a potential support against the common problem of foot pad dermatitis in poultry industry.
Layers
Study 7: Layer performance data from Study 7 are shown in table 9.
Treatment | Daily feed intake (g/hen/d) | Laying percentage (%) | Egg weight (g) | Albumin weight (g) | Yolk weight (g) | Shell weight (g) | Shell thickness (mm) | FCR per dozen eggs |
Control | 98.73 | 65.14 | 47.80 | 29.82 | 11.35 | 6.88a | 0.43 | 1.82 |
SCG 0.02% | 98.53 | 68.47 | 48.02 | 30.42 | 11.55 | 5.64b | 0.44 | 1.73 |
MCG 0.02% | 98.57 | 66.41 | 47.10 | 29.19 | 11.29 | 6.79a | 0.44 | 1.78 |
P-value | 0.776 | 0.147 | 0.966 | 0.527 | 0.978 | 0.0002 | 0.472 | - |
Table 9: Daily feed intake, egg, albumin, yolk and shell weights, shell thickness, and mortality for laying hens study 7.
a-c Means within a column without a common superscript differ (P<0.05).
Egg production parameters (hen egg production, henhouse egg production, and cumulative henhouse egg production) for hens fed both SCG and MCG at 0.02% were not different from control group (data not presented). There were no differences between feeding SCG or MCG at 0.02% compared to control group for some internal egg quality performance parameters of egg weight, albumen weight, yolk weight, and shell thickness. A difference was observed with a decrease in shell weight for eggs from layers fed SCG at 0.02% compared to control group.
Implications for layers: The reduced shell weight observed for layers fed SCG might be related to the numerical increase of egg production. Overall, although the results of this study did not show significant improvements with glucomannans included in the diets, the inclusion of SCG or MCG at 0.02% to the feed for 12 wks numerically increased the laying percentage (68.47% and 66.41%), respectively compared to control group (65.14%) (P=0.147). In addition, SCG or MCG also did not adversely affect overall egg quality, egg production, and mortality of layer hens suggesting being safe to be fed to laying hens at 0.02% of the diet.
A number of published studies were performed with prebiotics for laying hens with results showing either an improvement in egg laying performance and egg quality parameters [33,34], or no effects on these parameters [35,36]. Although majority of data evaluating prebiotics were performed in broilers, Corrigan et al. and Girgis et al. [37,38] demonstrated that supplementing diets with mannan-rich fraction increased microbiota diversity and reduced pathogen detection in ceca contents from laying hens. In addition, several studies also observed the effects of yeast glucomannans on reducing the negative effects of mycotoxins in laying hens [39,40].
In the current study, similar to other studies in the literature, we have observed numerical improvements but not significant differences, which is in alignment with previous findings from other authors. Therefore, considering the results from study 7 and literature data, feeding SCG or MCG up to 0.02% could be safe for laying hens.
Turkey hens
Study 8: Performance and mortality data for this study are presented in table 10. There were no differences in final BW, ADG, ADFI, and FCR comparing birds from treatment groups fed MCG at 0.20% or LCG at 0.20% of the diet compared to control group. However, there was a numerical improvement in these parameters by feeding MCG and LCG vs. control group on BW (2.60 kg and 2.58 kg vs. 2.56 kg; P = 0.396), ADG (59.87 g, 59.28 g vs. 58.56g ; P=0.415), and FCR (1.87 g:g, 1.87 g:g vs., 1.89 g:g; P=0.647), while ADFI was numerically increased by feeding SCG (112.17 g) vs. LCG (110.85 g) or control group (111.07 g) (P=0750).
Study 8 (7-48 days) | BW (kg) | ADG (g) | ADFI (g) | FCR (g:g) | Mortality (%) |
Control | 2.56 | 58.56 | 111.07 | 1.89 | 0.87 |
MCG 0.20% | 2.60 | 59.87 | 112.17 | 1.87 | 0.58 |
LCG 0.20% | 2.58 | 59.28 | 110.85 | 1.87 | 0.58 |
SEM | 0.018 | 0.425 | 1.598 | 0.019 | 0.415 |
p-value | 0.396 | 0.415 | 0.750 | 0.647 | 0.946 |
Study 9 (7-42 days) | BW (kg) | ADG (g) | ADFI (g) | FCR (g:g) | Mortality (%) |
Control | 2.05 | 49.37 | 98.58 | 2.00 | 0.61 |
MCG 0.20% | 2.06 | 49.29 | 95.83 | 1.94 | 0.91 |
SEM | 0.03 | 0.93 | 3.01 | 0.04 | 0.070 |
p-value | 0.281 | 0.322 | 0.684 | 0.329 | 0.401 |
Table 10: Growth performance and mortality data for turkey studies 8 and 9.
Mortality rate for all treatment groups was considered low for a study conducted under commercial conditions with average among treatment groups at 0.7% and with no differences among treatments (P>0.05).
The volcano plots showing individual comparisons between microbial profiles from dietary treatment with MCG at 0.20% in comparison with control group are shown in figures 1 and 2 from swab samples collected at d 30 and 48, respectively. Results from supplementing diets with LCG at 0.20% were not statistically significant (results not shown).
Figure 2: Effects of dietary supplementation with medium chain glucomannan at 0.20% on intestinal microbiota of turkey hens at 48 days of age.
At d 30, there was an increase in Lachnospiraceae and Bifidobacterium in cloacal swab samples from birds fed diets with MCG at 0.20% compared to control group. At d 48, similar results were observed for Lachnospiraceae and Bifidobacterium. However, Lactobacillus overgrowth was inhibited.
Study 9: Performance and mortality data for this study are listed in table 10. There were no significant differences in BW, ADG, ADFI, and FCR comparing turkeys fed diets with MCG at 0.20% to turkeys from control group. Similar to study 8, the mortality rate was low for all treatment groups with an average at 1.4% with no differences among treatment groups.
Implications for Turkeys: Although there were no significant improvements in growth performance of turkey poults under the trial conditions, feeding MCG at 0.20% resulted in numerical improvement compared to control group of ADG by 2.20%, increase in ADFI by 1%, and improvement in FCR by 0.75% (Study 9). These numerical differences in growth performance may be economically important in commercial conditions.
The current study evaluated similar glucomannans tested in the study from Flores et al. [14], however, there were no intentional stress applied in the current study, while stress challenge simulating transport conditions was applied by Flores et al. [14] at 29 d of age and resulted in MCG at 0.20% improving growth performance immediately after acute stress mainly driven by increased feed intake. In addition, glucomannans reduced the negative impact of stress by maintaining a stable intestinal microbiota by controlling the overgrowth of Lactobacillus and Fusobacteria while maintaining Bacteroidetes. At the end of the study (d 45), poults from control group and exposed to stress conditions recuperated the impaired performance. Therefore, glucomannans may benefit the growth performance of poults exposed to stress conditions and show greater effect immediately following the acute stress, which was not explored in the current studies 8 and 9. In the current study, there was an increase in Lachnospiraceae and Bifidobacterium in cloacal swab samples from birds fed diets with MCG at 0.20% compared to control group. Both Bifidobacterium and Lachnospiraceae are known as beneficial bacteria in poultry. Although in this study there were no differences in growth performance, it has been suggested that there is a correlation between Lachnospiraceae in the gut of birds with improved feed conversion which might be due to improvement in gut health and production of short chain fatty acids through degradation of plant materials [41]. In addition, Bifidobacteria and Lachnospiracea are commonly present in the large intestine, therefore, the increased relative abundance observed is an indication of earlier maturation of the gastrointestinal tract due to the development of the fermentative large intestine. These results on microbiota modulation are in agreement with Flores, et al. [14] testing similar glucomannans in turkey hens under acute challenge conditions.
Parks, et al. [42] evaluated the effects of MOS (0.01% to 6 wks, then 0.05% until 20 wks) in male turkey poults, which significantly increased 20-wk BW compared to control and significantly improved cumulative feed conversion from 0 to 3 wks, with no effects on mortality or cull rate. Similar positive results in feed conversion were observed in a 4-wk study with 1.00% MOS supplementation in female turkeys [43].
Other investigators have also established the safety of different MOS ingredients in turkeys. Devreese, et al. [44] found that 0.20% of a yeast-derived glucomannan mycotoxin adsorbent (GMA) in the diet for 12 wks overall did not adversely affect performance parameters in male turkeys fed control or Fusarium mycotoxin-contaminated diets, although mixed results were observed with the starter mycotoxin contaminated diets. Girish and colleagues [45,46] conducted two 12- wk studies in male turkeys. The findings in the first study were that feeding grains naturally contaminated with Fusarium mycotoxins and 0.20% GMA prevented decrease in duodenal villus height observed without GMA co-administration. In the second study, coadministration of 0.20% GMA in contaminated grains prevented the decreased body-weight gain caused by the contaminated diet during the grower and developer phases. Hooge [47] conducted a metaanalysis of turkey pen trials (1993-2003) with dietary MOS (BioMOS®; from the outer cell wall of Saccharomyces cerevisiae var. boulardii). Inclusion of MOS ranged from 0.10% continuous to 0.10% or 0.20% in step-down programs. The meta-analysis indicated that diets with MOS supported reduction in mortality and improved BW gain compared to a control diet.
Rahimi, et al. [48] investigated the effects of feeding 0.05% MOS for 21 d in female turkey poults challenged with Salmonella or Campylobacter. The MOS group had decreased levels of fecal Salmonella compared to the control group, with increased body weight and significantly increased intestinal villi surface area compared to controls. Ultrastructural analysis of the ileal tissue samples further supported the ileal mucosal benefits of MOS under the conditions of the study [49].
Literature and data from the current studies support that diets can be supplemented with MOS, including glucomannans MCG and LCG at the highest levels tested (0.20%), based on growth performance and mortality rate measured in turkeys. In addition, turkeys may benefit from the intestinal microbiota modulation provided by glucomannans on gut health, especially under challenging conditions. Further studies investigating the effects of glucomannans under stress conditions in turkey poults to the benefit on performance and intestinal microbiota of turkey hens under different trial conditions are recommended.
With the technology for producing glucomannans, distinct products were produced and tested. The results confirmed that the designed prototypes with different inclusion rates and chain lengths resulted in different physiological and performance effects. Therefore, specific glucomannans can be directed to different applications depending on the animal production conditions and the desired outcome (e.g., support feed intake and egg production, gut microbiota modulation, support bird welfare, etc.). However, it can also be said that in spite of chain length differences, glucomannans in general provide nutritional benefits that support and promote production parameters, nutritional health indicators, and microbiome resiliency.
Glucomannans were tested with different versions, use rates, and animal species in the current and previous studies. Based on the results with feeding glucomannans at use rates up to 2% with no adverse effects on growth performance, it can be inferred that glucomannans can be safely fed to poultry animals without adverse effects.
Although the trials discussed here give clear indications regarding the safe use of glucomannans at various use levels in multiple animal species, the ingredient produced from this technology should be further evaluated in varying conditions reflective of commercial production practices to be validated for potential practical use scenarios.
The authors thank Bruno Stengel, Delphine Melchior, Dirgam Roussan, Ibrahim Shaheen, Marijn Erinkveld, Alain Corniaux, Evelien van der Hoeven-Hangoor, Syrena Powell, for their assistance in supervising, conducting the animal trials, and reporting the results.
- Gibson GR, Roberfroid MB (1995) Dietary modulation of the human colonic microbiota: Introducing the concept of prebiotics. J Nutr 125: 1401-1412. [Ref.]
- Micciche AC, Foley SL, Pavlidis HO, McIntyre DR, Ricke SC (2018) A review of prebiotics against Salmonella in poultry: Current and future potential for microbiome research applications. Front Vet Sci 5: 1-11. [Ref.]
- Kim SA, Jang MJ, Kim SY, Yang Y, Pavlidis HO, et al. (2019) Potential for prebiotics as feed additives to limit foodborne Campylobacter establishment in the poultry gastrointestinal tract. Front Microbiol 10: 1-12. [Ref.]
- Meijerink N, de Oliveira JE, van Haarlem DA, Hosotani G, Lamot DM, et al. (2021) Glucose oligosaccharide and long-chain glucomannan feed additives induce enhanced activation of intraepithelial NK cells and relative abundance of commensal lactic acid bacteria in broiler chickens. Vet Sci 8: 110.
- Pourabedin M, Zhao X (2015) Prebiotics and gut microbiota in chickens. FEMS Microbiol Lett 362: fnv122. [Ref.]
- Meijerink N, Kers JG, Velkers FC, Van Haarlem DA, Lamot DM, et al. (2020) Early life inoculation with adult-derived microbiota accelerates maturation of intestinal microbiota and enhances NK cell activation in broiler chickens. Front Vet Sci 7: 584561. [Ref.]
- Liu N, Wang J, Liu Z, Wang Y, Wang J (2018) Effect of supplemental yeast cell walls on growth performance, gut mucosal glutathione pathway, proteolytic enzymes and transporters in growing broiler chickens. J Anim Sci 96:1330-1337. [Ref.]
- Song M, Fan Y, Su H, Ye J, Liu F, et al. (2019) Effects of Actigen, a second-generation mannan rich fraction, in antibiotics-free diets on growth performance, intestinal barrier functions and inflammation in weaned piglets. Livestock Sci 229: 4-12. [Ref.]
- Wang Y, Shen C, Huo K, Cai D, Zhao G (2021) Antioxidant activity of yeast mannans and their growth-promoting effect on Lactobacillus strains. Food & Function 12: 10423-10431. [Ref.]
- Markowiak P, Śliżewska K (2018) The role of probiotics, prebiotics and synbiotics in animal nutrition. Gut Pathog 10. [Ref.]
- Mohanty D, Misra S, Mohapatra S, Sahu PS (2018) Prebiotics and synbiotics: Recent concepts in nutrition. Food bioscience 26: 152- 160. [Ref.]
- Moreira LRS, Filho EXF (2008) An overview of mannan structure and mannan-degrading enzyme systems. Appl Microbiol Biotechnol 79:165-178. [Ref.]
- Flickinger EA, Fahey GG (2002) Pet food and feed applications of inulin, oligofructose and other oligosaccharides. Br J Nutr 87: S297-S300. [Ref.]
- Flores KR, Gernat AA, Fahrenholz A, de Oliveira JE, Hosotani G, et al. (2022) Effects of glucomannan feed additives on large white turkey female performance, gastrointestinal microbial population, and blood corticosterone levels in a simulated transport challenge. J Anim Sci Res 6. [Ref.]
- Meijerink N, de Oliveira JE, van Haarlem DA, Lamot DM, Velkers FC, et al. (2022) Long-chain glucomannan supplementation modulates immune responsiveness, as well as intestinal microbiota, and impacts infection of broiler chickens with Salmonella enterica serotype Enteritidis. Vet Res 53: 9. [Ref.]
- Wijtten PJA, Prak R, Lemme A, Langhout DJ (2004) Effect of different dietary ideal protein concentrations on broiler performance. Br Poult Sci 45: 504-511. [Ref.]
- Jana UK, Suryawanshi RK, Prajapati BP, Kango N (2021) Prebiotic mannooligosaccharides: Synthesis, characterization and bioactive properties. Food Chemistry 342: 128328. [Ref.]
- Faustino M, Durão J, Pereira CF, Pintado ME, Carvalho AP (2021) Mannans and mannan oligosaccharides (MOS) from Saccharomyces cerevisiae- A sustainable source of functional ingredients. Carbohydrate Polymers 272: 118467.[Ref.]
- Gomez B, Miguez B, Yanez R, Alonso JL (2017) Manufacture and properties of glucomannans and glucomannooligosaccharides derived from konjac and other sources. J Agric Food Chem 65: 2019- 2031. [Ref.]
- Orlean P (2012) Architecture and biosynthesis of the Saccharomyces cerevisiae cell wall. Genetics 192: 775-818. [Ref.]
- Spring P, Wenk C, Connolly A, Kiers A (2015) A review of 733 published trials on Bio-Mos®, a mannan oligosaccharide, and Actigen®, a second generation mannose rich fraction, on farm and companion animals. Journal of Applied Animal Nutrition 3(E8). [Ref.]
- Shashidhara RG, Devegowda G (2003) Effect of dietary mannan oligosaccharide on broiler breeder production traits and immunity. Poult Sci 82: 1319-1325. [Ref.]
- Baurhoo B, Goldflus F, Zhao X (2009) Purified cell wall of Saccharomyces cerevisiae increases protection against intestinal pathogens in broiler chickens. Int J Poult Sci 8:133-137. [Ref.]
- Koç F, Samli H, Okur A, Ozduven M, Akyurek H, et al. (2010) Effects of Saccharomyces cerevisiae and/or mannanoligosaccharide on performance, blood parameters and intestinal microbiota of broiler chicks. Bulgarian J Agri Sci 16: 643-650. [Ref.]
- Thomas WE, Nilsson LM, Forero M, Sokurenko EV, Vogel V (2004) Shear‐dependent ‘stick‐and‐roll’adhesion of type 1 fimbriated Escherichia coli. Molecular microbiology 53: 1545-1557. [Ref.]
- Chacher MFA, Kamran Z, Ahsan U, Ahmad S, Koutoulis KC, et al. (2017) Use of mannan oligosaccharide in broiler diets: an overview of underlying mechanisms. World’s Poult Sci J 73: 831-844. [Ref.]
- Baurhoo B, Phillip L, Ruiz-Feria CA (2007) Effects of purified lignin and mannan oligosaccharides on intestinal integrity and microbial populations in the ceca and litter of broiler chickens. Poult Sci 86: 1070-1078. [Ref.]
- Corrigan A, Horgan K, Clipson N, Murphy RA (2011) Effect of dietary supplementation with a Saccharomyces cerevisiae mannan oligosaccharide on the bacterial community structure of broiler cecal contents. Appl Environ Microbiol 77: 6653-6662. [Ref.]
- Kim GB, Seo YM, Kim CH, Paik IK (2011) Effect of dietary prebiotic supplementation on the performance, intestinal microflora, and immune response of broilers. Poult Sci 90: 75-82. [Ref.]
- Ao Z, Choct M (2013) Oligosaccharides affect performance and gut development of broiler chickens. Asian-Australas J Anim Sci 26: 116- 121. [Ref.]
- Rehman A, Arif M, Sajjad N, Al-Ghadi MQ, Alagawany M, et al. (2020) Dietary effects of probiotics and prebiotics on broiler performance, carcass and immunity. Poult Sci 99: 6946-6953. [Ref.]
- Van der Aar PJ, Molist FV, Van Der Klis JD (2017) The central role of intestinal health on the effect of feed additives on feed intake in swine and poultry. Animal feed science and technology 233: 64-75. [Ref.]
- Koiyama NTG, Utimi NBP, Santos BRL, Bonato MA, Barbalho R, et al. (2018) Effect of yeast cell wall supplementation in laying hen feed on economic viability, egg production, and egg quality. J Appl Poult Res 27: 116-123. [Ref.]
- Shalaei M, Hosseini SM, Zergani E (2014) Effect of different supplements on eggshell quality, some characteristics of gastrointestinal tract and performance of laying hens. Vet Res Forum 5: 277-286. [Ref.]
- Sacakli P, Ergun A, Koksal BH, Ozsoy B, Cantekin Z (2013) Effects of inactivated brewer’s yeast (Saccharomyces cereviciae) on egg production, serum antibody titres and cholesterol levels in laying hens. Veterinarija ir Zootechnika 61: 53-60.
- Yalçın S, Yalçın S, Onbaşılar İ, Eser H, Şahin A (2014) Effects of dietary yeast cell wall on performance, egg quality and humoral immune response in laying hens. Ankara Universitesi Veteriner Fakultesi Dergisi 61: 289-294. [Ref.]
- Corrigan A, Leigh RJ, Walsh F, Murphy R (2023) Microbial community diversity and structure in the cecum of laying hens with and without mannan-rich fraction supplementation. J Appl Poult Res 32: 100342. [Ref.]
- Girgis G, Powell M, Youssef M, Graugnard DE, King WD, et al. (2020) Effects of a mannan-rich yeast cell wall-derived preparation on cecal concentrations and tissue prevalence of Salmonella Enteritidis in layer chickens. Plos one 15: e0232088. [Ref.]
- Chowdhury SR, Smith TK (2004) Effects of feeding blends of grains naturally contaminated with Fusarium mycotoxins on performance and metabolism of laying hens. Poult Sci 83: 1849-1856. [Ref.]
- Rizzi L, Simioli M, Altafini A, Zaghini A (2005) Egg quality and mycotoxin residues of laying hens fed a diet containing Aflatoxin B1 and esterified glucomannans. Zootecnica International 6: 42-45.
- Stanley D, Hughes RJ, Geier MS, Moore RJ (2016) Bacteria within the gastrointestinal tract microbiota correlated with improved growth and feed conversion: challenges presented for the identification of performance enhancing probiotic bacteria. Front Microbio 7: 187. [Ref.]
- Parks CW, Grimes JL, Ferket PR, Fairchild AS (2001) The effect of mannanoligosaccharides, bambermycins, and virginiamycin on performance of large white male market turkeys. Poult Sci 80: 718- 723. [Ref.]
- Youssef IMI, Beineke A, Rohn K, Kamphues J (2012) Influences of increased levels of biotin, zinc or mannan-oligosaccharides in the diet on foot pad dermatitis in growing turkeys housed on dry and wet litter. J Anim Physiol Nutr 96: 747-761. [Ref.]
- Devreese M, Girgis GN, Tran ST, De Baere S, De Backer P, et al. (2014) The effects of feed-borne Fusarium mycotoxins and glucomannan in turkey poults based on specific and non-specific parameters. Food Chem Toxicol 63: 69-75. [Ref.]
- Girish CK, Smith TK (2008) Effects of feeding blends of grains naturally contaminated with Fusarium mycotoxins on small intestinal morphology of turkeys. Poult. Sci 87: 1075-1082. [Ref.]
- Girish CK, Smith TK, Boermans HJ, Karrow NA (2008) Effects of feeding blends of grains naturally contaminated with Fusarium mycotoxins on performance, hematology, metabolism, and immunocompetence of turkeys. Poult Sci 87: 421-432. [Ref.]
- Hooge DM (2004) Turkey pen trials with dietary mannan oligosaccharide: Meta-analysis, 1993-2003. Int J Poult Sci 3: 179- 188. [Ref.]
- Rahimi S, Kathariou S, Fletcher O, Grimes JL (2019) Effect of a direct-fed microbial and prebiotic on performance and intestinal histomorphology of turkey poults challenged with Salmonella and Campylobacter. Poult Sci 98: 6572–6578. [Ref.]
- Rahimi S, Kathariou S, Fletcher O, Grimes JL (2020) The effectiveness of a dietary direct-fed microbial and mannan oligosaccharide on ultrastructural changes of intestinal mucosa of turkey poults infected with Salmonella and Campylobacter. Poult Sci 99: 1135-1149. [Ref.]
Download Provisional PDF Here
Article Type: RESEARCH ARTICLE
Citation: de Oliveira J, Hosotani G (2023) Effects of Dietary Feeding Glucomannan on Broilers, Layers and Turkeys. J Anim Sci Res 7(2): dx.doi. org/10.16966/2576-6457.165
Copyright:© 2023 de Oliveira J, et al. This is an open-access article distributed under the terms of the Creative Commons Attribution License, which permits unrestricted use, distribution, and reproduction in any medium, provided the original author and source are credited.
Publication history:
SCI FORSCHEN JOURNALS
All Sci Forschen Journals are Open Access