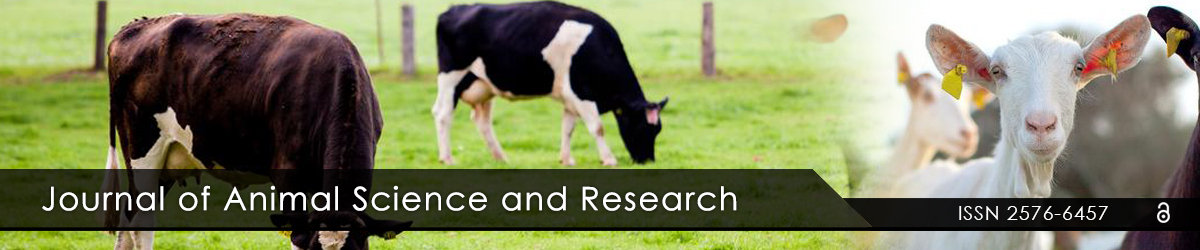
Full Text
JPS Neel* KE Turner SW Coleman MA Brown PH Gowda JL Steiner
Grazing lands Research Laboratory-PA-ARS-USDA, 7207 West Cheyenne Street, El Reno, Oklahoma, USA*Corresponding author: JPS Neel, Grazing lands Research Laboratory-PA-ARS-USDA, 7207 West Cheyenne Street, El Reno, Oklahoma, 73036, USA, E-mail: Jim.Neel@ars.usda.gov
Methane (CH4 ) gas has been suggested as a major contributor to global warming. Increased rumen fermentation CH4 is associated with high fiber diets and its production is inversely related to energy availability for the host animal. Within the US cow-calf sector, cattle diet ingredients are primarily high fiber feeds (e.g. pasture forages and hay) which cannot be utilized as human food. It is suggested the primary U.S. beef production model has led to the selection of an animal type which is efficient within the feedlot finishing sector, but not within the cow-calf sector due to increased cow size. We examined the relationship between cow frame size (FS), enteric CH4 production, and cow productivity within a tall-grass prairie grazing system. Twenty-eight lactating Angus cows (BW: 545 ± 49 kg) of either medium FS (n=14) or large FS (n=14) were utilized in this study. Animals grazed simultaneously within a pasture in central Oklahoma, USA during the summer season (SS). Daily estimates of individual animal enteric CH4 and carbon dioxide (CO2 ) production were taken via commercially available breath analyzers deployed within the pasture. Medium-frame cows had a lower body weight (BW) and frame score than large FS (481 versus 609 kg, and 4.6 versus 6.8, respectively). Large-frame cows produced greater daily CH4 (315 versus 270 g/day) compared to medium-frame cows. There was no difference in SS enteric CH4 per unit of 205d adjusted calf weaning weight (WW; 0.11 kg/kg).When expressed as CH4 per unit of cow BW for the total SS, large frame cows produced less CH4 (48.3 versus 52.6 g/kg BW). Based on estimated dry matter intake (DMI), large-frame cows required less herbage dry matter per unit of 205 day WW than medium (8.4 versus 9.4 kg DM/kg WW), and produced greater CH4 per unit of DMI (13.6 versus 12.2 g/kg).Our results indicate large-frame cows may have increased rumen DM digestion, which results in greater methane production per unit of DMI, and less DM required per unit of calf production. Therefore, within the southern Great Plains, large-frame cows appear to be more efficient at converting native prairie into saleable product, and cow efficiency has not been sacrificed due to increased cow size. The sector’s methane footprint may best be improved through rumen fermentation manipulation.
Methane; Beef cows; Pasture; Frame size
Methane (CH4 ) gas has been suggested as a major contributor to global warming. Within the USA, all agriculture (livestock, agricultural soils, and rice production) contributed about 9% to total greenhouse gas emissions in 2015 (www.epa.gov/ghgemissions/ sources-greenhouse-gas-emissions). In ruminants, enteric CH4 is a necessary byproduct of fermentation within the symbiotic relationship between rumen microbes and the ruminant host. Rumen microbial fermentation provides the animal with both maintenance and production energy. The continual flow of microbes out of the rumen into the lower gastrointestinal tract also provides a source of high-quality protein to the animal. To ensure the availability of energy and protein, the host provides the microbial population with a safe and controlled anaerobic environment, a continual source of fermentation substrate, and the removal of fermentation end-products [1].
Primary rumen fermentation end products are the VFAs acetate, propionate, and butyrate, as well as carbon dioxide (CO2 ), hydrogen (H2 ), CH4 and ammonia (NH3 ). All of these end-products must be removed from the rumen to ensure a continuation of the fermentation process [1]. The VFAs are either absorbed through the rumen wall (providing the host ruminant with maintenance and production energy) or flow out into the lower tract [2]. Carbon dioxide and H2 are the natural result of carbohydrate fermentation as the microbes attain their energy needs. Methane is then produced by microbial reactions combining CO2 and H2 , and this process is essential to ensure that H2 doesn’t accumulate to the point of preventing continued ruminal fermentation [3]. The gaseous end products are removed from the rumen through eructation by the animal [1].
During ruminal fermentation of forages, acetate is the primary VFA produced and its production results in greater production of CH4 compared to the secondary VFA propionate. Any shift from microbial production of acetate to propionate therefore results in less CH4 production per unit of feed, increased fermentation efficiency, and greater energy availability to the host animal (given equivalent dilution and passage rates; [4]. This in turn leads to improved animal production efficiency. Factors which influence CH4 production in the rumen are: level of feed intake, type of carbohydrate in the diet, feed processing, addition of lipids or ionophores to the diet, and alterations in the ruminal microflora [5].
Within the beef industry, about 70% of the feedstuffs utilized as a whole are consumed by the cow-calf sector [6]. It is important to realize that within the cow-calf sector, these feedstuffs are primarily comprised of high fiber pasture, forage, roughage, and agricultural industry by-products. Worldwide, ruminant feed utilized in the production of high biological value human food and fiber is made up of an even higher percentage of fibrous feedstuffs and most of those feedstuffs are produced on land that is unsuitable for crop/human food production [7]. These renewable fibrous feedstuffs do not provide food and fiber to humans unless they are first processed by rumen microbes. The prediction of an ever-increasing global population and improved wealth within that population will lead to an increased demand for ruminant food and fiber products [8]. This increased demand makes continued improvement in the efficiency of use of ruminant feedstuffs essential.
The most efficient cow size for use in grazing systems has long been debated. It is often suggested that the primary U.S. beef production model (a shift to larger frame cattle and larger carcasses) has led to the selection of an animal type which is efficient for beef production within the feedlot-concentrate based finishing sector, but also led to sacrificed production efficiency within the cow-calf sector due to increased cow size and their inability to achieve adequate dry matter intake (DMI) of fibrous feedstuffs to meet added nutritive requirements for maintenance and production. Since increased rumen CH4 production is inversely related to energy availability and production efficiency for the host animal, the goal of our research was to determine the relationship between cow frame size (FS; medium = 3.5 to 5.7 frame score, large = > 5.7 frame score), enteric CH4 production, and cow productivity for lactating Angus cows grazing native tall-grass prairie during the summer season (SS) in central Oklahoma, USA.
Research use of animals in this study was conducted under an approved protocol by the Institutional Animal Care and Use Committee, Grazing lands Research Laboratory, El Reno, OK, USA.
A 64-ha native grassland pasture growing on a Norge silt loam (fine-silty, mixed, active, thermic Udic Paleustolls) at the Grazing lands Research Laboratory was used. This pasture is one of four contiguous, like sized, native pastures that are rotationally grazed over 12 months by a cow herd of 50 Angus cows. This stocking rate would be considered typical for native range within the region, when wanting to ensure adequate forage availability during years of below average precipitation. The predominant grass species in the mixed sward was big bluestem (Andropogon gerardii Vitman); other minor species were: little bluestem [Schizachyrium scoparium (Michx.) Nash], indian grass [Sorghastrum nutans (L.) Nash], switch grass (Panicum virgatum L.), numerous forbs and cool-season grasses.
For CH4 and CO2 measurements, 28 Angus cows (BW: 545 ± 49 kg) of either medium-FS (n=14) or large-FS (n=14), and their suckling calves, were utilized in this study. Cow FS was generated from individual hip heights [9]. Each cow type was bred to bulls of similar type (e.g. medium FS bulls to medium FS cows). At weaning, individual calf weights were taken, and cow body condition scores (BCS) were taken throughout the year. Cow BCS score was based on a 1-9 scoring system, with a score of 5-6 being desirable, 1-4 being too thin and 7-9 being overly fat. All animals grazed simultaneously within a native tall-grass prairie pasture in central Oklahoma, USA during the summer season (SS). Summer season designation was based on the summer solstice, and the fall equinox. All calves were born during the period of March through early April 2014 and were weaned on 24 September 2014. For productivity comparison between cow frame types, calf weaning weights (WW) were adjusted to 205-day equivalents [10] due to all calves being weaned on the same day.
Throughout the study period, daily estimates of individual animal enteric CH4 and CO2 production were taken via a commercially available breath analyzer, with two Green Feed (C-Lock, Inc., Rapid City, SD) systems deployed continually in the pasture throughout each day and during the entire SS. All cows could access the samplers at any time during a daily 24 hour period, with up to 8 visits per day. The systems are automated emissions measuring devices that sample exhaled breaths and eructation when trained cows insert their heads in a hood to retrieve a pelleted feed treat. Each cow utilized for CO2 and CH4 measurement was fitted with a unique electronic identification tag. When an individual animal put its head in the breath-analyzing area, the unit identified the animal and dispensed a small amount feed (approximately 90 grams total, given in 3 portions) in order to insure the animal remains engaged with the Green Feed unit for sufficient time (approximately 3 to 8 minutes total per sampling) to conduct a reading. After the reading is taken, the animal is not allowed more feed over the next 3 hours; this restriction period allows other cows to be sampled. During the data collection period, data were remotely uploaded and algorithms applied by the manufacturer to calculate mean individual cow daily values. Initially, 8 cows from the herd were trained to utilize two breathe analyzer units within a small pen. The 8 “trainer” cows and two Green Feed systems were then introduced into the pasture with the main herd, to teach their herd-mates how to utilize the units. The whole herd was trained for 30 days prior to study data collection. Of a total 50 cow herd, 28 head utilized the systems a sufficient amount over the data collection period (June Solstice through September Equinox, 93d) to provide usable estimates of CH4 and CO2 production. A criterion used to designate a “sufficient amount” of unit utilization was based on the work of Arthur et al. (2017) [11]. Those researchers reported that over an experimental period, a minimum of 30 individual animal records (with a minimum visit duration time of 3 minutes) was required. Beyond 30 individual animal visits there was no substantial reduction in variance. They suggested that the focus during a research study should not be the number of daily individual animal visits, but attaining the suggested minimum number of visits (30) and that the events were evenly distributed across the experimental period. In our study, the total number of emissions visits used in the data set was 4,474. The average number of usable visits per animal in the SS study period was 93 per animal. Considering the variability in the spot samples and the number of visits, the averaged 95% CI in the individual animal averaged value for the SS period was +8.3%. We estimate, the herd averaged methane emissions value for each group during the summer period to have a 95% CI of between 2 to 7%.
For herbage nutritional value estimation, six rumen-cannulated beef steers were used to obtain samples via the rumen evacuation technique outlined by Lesperance et al. (1960) [12]. After initial rumen clean out, the steers were allowed to graze for approximately 45 minutes and then returned to the chute in order to collect a sample of grazed forage from the rumen through the cannula. Sampling times were 24 June and 18 July in 2014. This time of year represents a period of high nutritive value (June/July) for tall-grass native prairie in central Oklahoma. Rumen samples were rinsed with tap water to help minimize contaminants on forage collected from the rumen environment which would alter forage nutritive value estimates (e.g. microbes, remaining rumen fluid, animal saliva), frozen at –20°C, then freeze-dried. Dried samples were ground to pass a 2-mm screen in a Wiley mill (model #4, Arthur H. Thomas Co., Philadelphia, PA, USA) and then used to determine: Dry matter(DM) [13]; in vitro true digestibility (IVTD) using procedures for a Daisy incubator (Ankom Technology Corp., Fairport, NY, USA) with McDougall’s buffer [14]; total N (Nitrogen Analyzer, Elementar Vario MACRO, Elementar Americas Inc., Mount Laurel, NJ, USA); and neutral detergent fiber (NDF) and acid detergent fiber (ADF) [15,16] using a batch processor (Ankom Technology Corp., Fairport, NY, USA). For the IVTD procedure, rumen fluid was collected from two ruminally cannulated beef steers maintained on Bermuda grass [Cynodon dactylon (L.) Pers.] pasture and offered native warm-season grass hay [predominately big bluestem (Andropogon gerardii Vitman)]. Total N was converted to crude protein (CP) using the following relationship: %CP = % Total N multiplied by 6.25.
Dry matter intake (DMI) estimates were calculated using the following equation: Intake = [(Fecal Output) / (1 – herbage digestibility)]. Fecal output was estimated utilizing 8 cows (4 mediumand 4 large-FS) randomly selected from the group of 28 cows utilized for CH4 and CO2 estimates. These 8 cows were dosed with chromic oxide (Cr2 O3 ) rumen boluses (payout = 1.42 g/day; (Captec Ltd., Auckland, NZ)). Samples of voided feces were collected morning and late afternoon from each cow for 5 days. Collected samples were freeze dried, and ground to pass a 1-mm screen in an Udy cyclone mill (Udy Corporation, Fort Collins, CO, USA). Duplicate 0.20 g fecal samples were oven dried at 100°C and dry weights obtained. Oven-dry samples were then dry-ashed at 450°C for 48 hours and the ashed residues were subject to the modified dry ash procedure outlined by [17] for Cr extraction in preparation for analysis for Cr using Inductively Coupled Plasma Optical Emission Spectrometry on a Spectro Genesis ICP (Spectro Analytical Instruments GmbH, Kleve, Germany). Fecal output estimates were then used in conjunction with estimated forage indigestibility to calculate estimated DMI for each cow (as kg d-1 and% of body weight). The mean value within FS was then used for calculation of DMI for each cow utilized in the collection of enteric CH4 and CO2 data.
Statistical analyses
Cow and calf data were analyzed as a completely randomized design with the GLM procedure (SAS Inst. Inc., Cary, NC). The linear model included cow frame score (fixed) and a random residual. Treatment least squares means were calculated, and means were compared using PDIFF when protected by a significant (P <0.05) F-value. Cow was used as the experimental unit.
Nutritive value of clipped and rumen evacuated herbage is presented in table 1. Herbage samples obtained from rumencannulated steers via rumen evacuation had greater (P<0.05) CP content and lower (P<0.05) NDF and ADF versus clipped samples during both collection times. Sample IVTD values were similar across sampling types and times except for 18 July where IVTD was lower (P < 0.05) for the clipped sample. These findings are as expected based on pasture maturation over time and the animal’s ability to overcome decreasing pasture quality through selective grazing. Results also confirm that rumen evacuation is the superior method of assessing pasture nutritive value compared to clipped samples, especially under our study’s conditions of rapid pasture maturation. Dry matter intake estimates were determined, given the need for DMI estimates to be representative of study environmental conditions, diet quality, and animal physiological state [18] by utilizing the indigestible marker method (Cr2 O3 ). Lower IVTD estimates for the 18 July sampling date of the clipped sample shows that DMI estimates would be substantially lower for clipped samples versus that generated by animal selected herbage. Over the 25-day period, available herbage biomass would not have limited cow DMI, and herbage nutritive value would not be limiting in terms of lactating cows 3-4 months post calving, having a peak milk production of 9.1 kg/day, and weighing up to 635 kg [19].
Collection date | ||||||
Nutritive Value | 24-Jun-14 | 18-Jul-14 | Overall Mean | |||
Attribute1 | Value SE | Value SE | ||||
CP, | Rumen Evacuated | 12.5a | 0.53 | 10.8b | 0.53 | 11.7 |
% | Hand Clipped | 6.0c | 0.37 | 6.2c | 0.37 | 6.1 |
NDF, | Rumen Evacuated | 65.0d | 1.25 | 70.5c | 0.93 | 67.3 |
% | Hand Clipped | 77.9a | 0.89 | 74.4b | 0.89 | 76.1 |
ADF, | Rumen Evacuated | 33.3d | 1.3 | 38.9c | 1.3 | 36.1 |
% | Hand Clipped | 43.9a | 0.92 | 41.8b | 0.92 | 42.8 |
IVTD, | Rumen Evacuated | 69.7a | 1.88 | 65.8a | 1.88 | 67.7 |
% | Hand Clipped | 66.9a | 1.32 | 54.8b | 1.32 | 60.8 |
Table 1: Dry matter concentrations of crude protein (CP), neutral
detergent fiber (NDF), acid detergent fiber (ADF), and in vitro true
digestibility (IVTD) of rumen evacuated and hand clipped plant samples
collected from native pasture during summer.
1Values within nutritive value attribute and across collection dates with unlike superscripts differ (P<0.05).
Table 2 presents cow and calf attributes. Medium-frame cows had a lower (P<0.001) BW (481 versus 609 kg), and FS (4.6 versus 6.8) than large-frame cows. This was expected due to experimental design. Cow body condition scores (BCSs) were taken in mid-winter, earlyspring, early-summer and fall during 2014. Cow BCSs did not differ between cow frame-type at any scoring time, and this suggests there is no difference between frame-type in their ability to extract energy from available forage, or to metabolically process and allocate it. Both cow types had their numerically lowest BCS corresponding to earlysummer, this reflects their need to utilize body fat reserves post calving during their peak milk production period, prior to the return of high quality warm season pasture. However, by fall their BCSs reflect that they had already begun replenishing those stores. Estimated DMI did not differ between cow types, although large-frame cows had numerically greater daily and summer season (SS) DMIs (22.2 versus 23.2 kg, and 2069 versus 2158 kg). These results reflect the greater estimate of DMI as a percent of BW for medium-frame cows as determined through the use of the indigestible marker method (4.63 versus 3.81%). Clearly the high DMI estimate for both cow types reflects the drive for DMI due to their stage of milk production, as well as the quality of the herbage [20] showed that frame size was positively related to cow DMI on pasture, but their R2 values ranged from 0.46 to 0.74, with the lower correlations associated with higher forage quality during the earlier part of the grazing season. Their lowest correlation values were associated with spring grazing on cool-season pastures during the early and rapid growth period, when pasture was at its highest quality. For warm season grasses within our plant growth zone [21], this same period of rapid early growth and highest quality relates to early- to mid-summer. Large-frame cows produced a heavier (P<0.01) calf than medium-frame cows (261 versus 222 kg). When expressed in terms of kg of total SS DMI per unit of calf weight, large frame cows required less (P<0.05) DM per unit of WW. Large frame cows consumed an estimated 8.4 kg of herbage DM per unit of weaned calf while their medium framed counterparts required 9.4 kg.
Cow Type | ||||
Animal Attribute1 | Medium Frame | Large Frame | SE | P value |
BW, kg | 481b | 609a | 13.1 | <0.0001 |
Frame Score2 | 4.6b | 6.8a | 0.18 | <0.0001 |
Cow BCS, Winter 2014 | 5.6 | 5.6 | 0.14 | 0.7213 |
Cow BCS, Spring 2014 | 5.5 | 5.2 | 0.13 | 0.1759 |
Cow BCS, Summer 2014 | 4.7 | 4.9 | 0.18 | 0.681 |
Cow BCS, Fall 2014 | 5.4 | 5.3 | 0.21 | 0.8109 |
Cow DMI, kg/d | 22.2 | 23.2 | 0.57 | 0.2439 |
SS DMI, kg | 2069 | 2158 | 52.7 | 0.2439 |
205d WW, kg | 222b | 261a | 8.6 | 0.0041 |
SS DMI / WW, kg/kg | 9.4a | 8.4b | 0.31 | 0.0324 |
Table 2: Mean cow body weight (BW), frame score, body condition score
(BCS), daily, and summer season (SS; based on summer solstice to fall
equinox) estimated cow dry matter intake (DMI), calf 205 day adjusted
weaning weight (WW) and SS DMI per unit of WW by cow type.
1 Values within animal attribute and across cow type with unlike superscripts differ (see P value within table line).
Cow enteric CH4 and CO2 and their relationship to estimated DMI and production are presented in table 3. Large-frame cows produced greater (P<0.001) daily CH4 (315 versus 270 g/d) and CO2 (9658 versus 8376 g/d) compared to medium-frame cows. In addition, when expressed as CH4 and CO2 per unit of DMI, large-frame cows produced greater (P<0.01) CH4 (13.6 versus 12.2 g/kg) and CO2 (417 versus 378 g/kg). Greater production of these gaseous fractions without increased DMI could be possible if: 1) medium-frame cows are experiencing increased propionate production relative to acetate per unit of DMI fermented, resulting in reduced CH4 and CO2 production; or 2) if we assume similar end-product ratios per unit of DMI fermented between cow FS types, large-frame cows are experiencing increased microbial digestion of DM per unit of DMI, resulting in increased CH4 and CO2 production. The second scenario presented seems reasonable as both cow types are pastured together, resulting in the same forage being on-offer to both cow types. Given there was no difference in estimated DMI between cow FS types, this increased CH4 and CO2 per unit of DMI seems to indicate that large-frame animals may be capable of improved DM fermentation efficiency. De Mulder et al. (2018) [22] reported that Belgian Blue heifers were more efficient than Holstein Friesian heifers of the same age, stage of gestation, and fed the same diet, based on the ratio of enteric CH4 to CO2 on a mole per mole basis. In their study, the numerically heavier Belgian Blue heifers (594 ± 42 versus 558 ± 39 kg) had lower (P<0.01) DMIs and tended to have greater (P=0.05) enteric CH4 production per unit of DMI. The improved efficiency of the Belgian Blue versus the Holstein Friesian heifers was attributed to more efficient microbial fermentation of feed by the Belgian Blue heifers. The results of De Mulder et al. (2018) is in agreement with ours in that although estimated DMI did not differ between cow types, CH4 per unit of DMI was greater for large-FS than medium-FS cows. Improved microbial fermentation efficiency could be due to increased DM rumen retention time for large frame cows. Huhtanen et al. (2016) [23] showed an increase in CH4 per unit of DMI associated with increased retention times based on the modeling of rumen function. They indicated that this increase in CH4 per unit of DMI was partly associated with enhanced organic matter digestibility within the rumen, and also with reduced efficiency of microbial cell synthesis. Bacterial CP synthesis efficiency is reduced by increased mean rumen retention time (MRT) due to more digestible energy being required for microbial population maintenance [19]. Huhtanen, et al. (2016) [23] suggested that this decreased efficiency results in less ability for the microbial population to serve as a H2 sink, resulting in relatively more H2 being shunted into CH4 production. Increased MRT is associated with lower intake level, higher fiber level in the diet, and physical form of the diet [24]. The lack of difference in estimated DMI between cow FS types in our study, and the expected greater rumen capacity for the large-FS cows would lessen the impact of intake on MRT, resulting in an expected increase in MRT and rumen DM digestion. Since the more readily fermentable and digestible portion of the forage consumed by the cows in our study would necessarily be fermented first, an increase in MRT would result in greater a greater proportion of dietary cellulose being digested in the rumen. Greater ruminal cellulose digestion would lead to increased acetate and CH4 production [24], which in turn would result increased acetate and CH4 production per unit of DMI. It is therefore plausible for the large-FS cows in our study to have greater daily CH4 production without having a higher DMI. More research is needed with grazing livestock in this area to further verify and illuminate the cause(s) and implications of our findings.
Cow Type | ||||
Animal Attribute1 | Medium Frame | Large Frame | SE | P value |
Cow CH4, g/d | 270b | 315a | 7.6 | 0.0003 |
Cow CO2, g/d | 8376b | 9658a | 188 | <0.0001 |
CH4 / DMI, g/kg | 12.2b | 13.6a | 0.36 | 0.0097 |
CO2 / DMI, g/kg | 378b | 417a | 8.7 | 0.0037 |
SS Cow CH4, kg | 25.1b | 29.3a | 0.71 | 0.0003 |
SS Cow CO2, kg | 779b | 898a | 17.6 | <0.0001 |
SS CH4 / BW, g/kg | 52.6a | 48.3b | 1.44 | 0.0447 |
SS CO2 / BW, kg/kg | 1.63a | 1.48b | 0.0348 | 0.0052 |
SS CH4 / WW, kg/kg | 0.114 | 0.114 | 0.0047 | 0.9891 |
SS CO2 / WW, kg/kg | 3.55 | 3.51 | 0.145 | 0.8478 |
Table 3: Mean daily and summer season (SS), based on summer solstice to fall equinox) enteric methane (CH4) and carbon dioxide (CO2), and their
relationship to cow body weight (BW), dry matter intake (DMI), and calf 205 day adjusted weaning weight (WW) by cow type for.
1Values within animal attribute and across cow type with unlike superscripts differ (see P value within table line).
Medium-FS cows produced less (P<0.001) CH4 and CO2 over the SS (25.1 versus 29.3 kg, and 779 versus 898 kg, respectively), reflecting their lower daily CH4 and CO2 emissions (Table 3). However, when expressed as CH4 and CO2 per unit of cow BW for the SS, large-frame cows produced less (P<0.05) CH4 and CO2 (48.3 versus 52.6 g/kg BW, and 1.48 versus 1.60 kg kg-1 BW, respectively). Reduced SS enteric gas production per unit of BW may indicate lower maintenance requirements on pasture per unit of BW for large-frame cows during the SS. Although they did not specifically calculate it in their paper, calculations based on the presented data in [22] showed a numerically greater production of CH4 per unit of body weight for the less efficient and lower BW HF versus the Belgium Blue heifers.
One of the main factors by which the efficiency of productivity in brood cows is evaluated is in relation to calf weaning weight. As previously presented (Table 2), large-frame cows produced on average a 39 kg heavier calf on the same amount of DMI. When estimated SS DMI was divided by 205 d WW, it showed that1 kg less DM per unit of WW was required by large-frame cows. Summer season enteric CH4 and CO2 emissions per unit of adjusted WW are presented in table 3. There was no difference between cow FS types for either enteric CH4 (0.11 kg/kg) or CO2 (3.5 kg/kg) production over the entire SS when expressed on a per-unit of calf WW basis. From the stand point of enteric CH4 and CO2 , our results indicate there is no advantage for reduced frame size cows regarding enteric gas emissions relative to amount of product produced, at least when available herbage and nutritive value is not limiting given cow stage of production. Further improvement in production efficiency within the southern Great Plains may best be achieved through manipulation of rumen fermentation. This process should aim to create a shift away from acetate production towards more propionate. Such a shift would result in less methane production per unit of DM and greater production energy availability to the animal [26]. In addition to the known advantage of supplemental monensin in reducing rumen methane production, promising novel approaches have also been reported [26,27].
Large-frame cows weighed 128 kg more than medium-framed cows, they produced a 39 kg heavier calf at weaning, and there was no difference in estimated DMI, or BCS (measured over the entire year) between cow FS types. Based on estimated SS DMI, our research results indicate that large-frame cows may be more efficient at converting central Oklahoma native tall-grass prairie into saleable product. Large-frame cows produced more enteric CH4 and CO2 over the SS than medium-frame, but less per unit of BW, which suggests they may have improved energy use efficiency. Although large-frame cows produced more CH4 per unit of estimated DMI, there was no difference between cow FS type for DMI.
Our results indicate large-frame cows may have increased rumen DM digestion, resulting in greater methane production per unit of DMI. The improved digestion appears to result in less DM required per unit of calf production. Therefore, within the southern Great Plains, large-frame cows appear to be more efficient at converting native prairie into saleable product, and cow efficiency has not been sacrificed due to increased cow size. The sector’s methane footprint may best be improved through rumen fermentation manipulation.
Improved rumen fermentation digestion could be due in part or total to either increased MRT, or differences in the rumen microbiome. When enteric gas production over the SS is related to weight of calf produced, there was no difference between cow type in the amount of cow CH4 or CO2 produced per kg of calf. The cow-calf sector’s methane footprint may best be improved through rumen fermentation manipulation. Certainly this area of research is deserving of future efforts, due to the implications of our experimental results, and to provide insight into yearlong variability due to season.
The authors wish to thank Scott Schmidt, Clendon Tucker, Nelson Reese, Craig Mittelstaedt, and Cindy Coy for their technical support. This research did not receive any outside or specific funding.
The authors declare no conflicts of interest.
- Hungate RE (1988) Introduction: The ruminant and the rumen. In: The rumen microbial ecosystem. Hobson PN (Ed), Elsevier Science Publishing Company, Inc., New York, USA, 1-19.
- Dijkstra J, Forbes JM, France J (2005) Introduction. In: Quantitative aspects of ruminant digestion and metabolism (2nd Edn). DijkstraJ, Forbes JM France J (Eds). CABI Publishing, United Kingdom and Cambridge, MA, USA, 1-12.
- Wolin WJ, MillerTL (1988) Microbe-microbe interactions. In: The rumen microbial ecosystem. PN Hobson (ed) Elsevier Science Publishing Company, Inc., New York, USA, 343-359.
- Russell JB, Wallace RJ (1988) Energy yielding and consuming reactions. In: The rumen microbial ecosystem. PN Hobson (Ed) Elsevier Science Publishing Company, New York, USA, 185-215.
- Van Nevel CJ, Demeyer DI (1988) Manipulation of rumen fermentation. In: The rumen microbial ecosystem. PN Hobson (ed) Elsevier Applied Science, London and New York, USA, 387-443.
- Shike DW (2013) Beef cattle feed efficiency. University of Illinois. Driftless Region Beef Conference 2013. USA. [Ref.]
- Mottet A, de Haan C, Falcucci A, Tempio G, Opio C, et al. (2017) Livestock: On our plates or eating at our table? A new analysis of the feed/food debate. Global Food Security 14: 1-8. [Ref.]
- Food and Agriculture Organization of the United Nations (2009) The State of Food and Agriculture – Livestock in the balance. Rome, ISBN 978-92-5-106215-9. [Ref.]
- Dolezal SL, Coe N (1996) Hip height and frame score determination. Oklahoma Cooperative Extension Fact Sheet. ANSI-3271. http:// osufacts.okstate.edu [Ref.]
- Taylor RE (1994) Beef production and management decisions. (2nd Ed.) S Walvoord (ed). MacMillan Publishing Company, New York, USA. [Ref.]
- Arthur PF, Barchia IM, Weber C, Bird-Gardiner T, Donoghue KA, et al. (2017) Optimizing test procedures for estimating daily methane and carbon dioxide emissions in cattle using short-term breath measures. J Anim Sci 95: 645-656. [Ref.]
- Lesperance AL, Bohman VR, Marble DW (1960) Development of techniques for evaluating grazed forage. J. Dairy Sci 43: 682-689. [Ref.]
- AOAC-Association of Official Analytical Chemists (1999) Official Methods of Analysis, 16th ed. Washington, DC, USA.
- McDougall EI (1948) Studies on ruminant saliva. I. Composition and output of sheep saliva. Biochem J 43: 99-109.[Ref.]
- Goering HK, Van Soest PJ (1970) Forage fiber analyses (apparatus, reagents, procedures, and some applications). USDA-ARS Agricultural, Washington, DC. [Ref.]
- Van Soest PJ, Robertson JB, Lewis BA (1991) Methods of dietary fiber, neutral detergent fiber, and nonstarch polysaccharides in relation to animal nutrition. J Dairy Sci 74: 3583-3597. [Ref.]
- Enders A, Lehmann J (2012) Comparison of wet-digestion and dryashing methods for total elemental analysis of biochar. Commun Soil Sci Plant Anal 43: 1042-1052. [Ref.]
- Coleman SW, Gunter SA, Sprinkle JE, Neel JPS (2014) Beef Species Symposium: Difficulties associated with predicting forage intake by grazing beef cows. J Anim Sci 92: 2775-2784. [Ref.]
- NRC (2000) Nutrient requirements of beef cattle (7th Ed.) 1996. Washington, DC, USA: National Academy of Science and National Research Council. [Ref.]
- Holloway JW, Butts Jr. WT (1984) Influence of cow frame size and fatness on seasonal patterns of forage intake, performance and efficiency of Angus cow-calf pairs grazing Fescue-legume of Fescue pastures. J Anim Sci 59: 1411-1422. [Ref.]
- Ball DM, Hoveland CS, Lacefield GD (1996) Sothern Forages (2nd Ed). Potash & Phosphate Institute, Norcross, GA, USA 18-27. [Ref.]
- De Mulder T, Peirena N, Vandaelea L, Ruttinkb T, De Campeneerea S, et al. (2018) Impact of breed on the rumen microbial community composition and methane emission of Holstein Friesian and Belgian Blue heifers. Livestock Sci 207: 38-44. [Ref.]
- Huhtanen P, Ramin M, Cabezas-Garcia EH (2016) Effects of ruminal digesta retention time on methane emissions: a modelling approach. Anim Production Sci 56: 501-506. [Ref.]
- Faichney GJ (2005) Digesta flow. In: Quantitative aspects of ruminant digestion and metabolism (2nd Ed) Dijkstra J, Forbes JM, France J (Ed). CABI Publishing, United Kingdom and Cambridge, MA, USA, 49-86. [Ref.]
- Fahey Jr. GC, Berger LL (1988) Carbohydrate nutrition of ruminants. In: The ruminant animal- Digestive physiology and nutrition. Church DC (Ed). Prentice Hall, Englewood Cliffs, New Jersey, USA, 269-297.
- Soltan YA, Hashem NM, Morsy AS, El-Azrak KM, Nour El-Din A, et al. (2019) Comparative effects of Moringa oleifera root bark and monensin supplementations on ruminal fermentation, nutrient digestibility and growth performance of growing lambs. Anim Feed Sci Technol 235: 189-201. [Ref.]
- Soliva CR, Hindrichsen IK, Meile L, Kreuzer M, Machmuller A (2003) Effects of mixtures of lauric and myristic acid on rumen methanogens and methanogenesis in vitro. Lett Appl Microbiol 37: 35-39. [Ref.]
Download Provisional PDF Here
Article Type: RESEARCH ARTICLE
Citation: Neel JPS, Turner KE, Coleman SW, Brown MA, Gowda PH, et al. (2019) Effect of Frame Size on Enteric Methane (CH4 ) and Carbon Dioxide (CO2 ) Production by Lactating Beef Cows Grazing Native Tall-Grass Prairie Pasture in Central Oklahoma, USA, 1: Summer Season. J Anim Sci Res 3(3): dx.doi.org/10.16966/2576-6457.130
Copyright: © 2019 Neel JPS, et al. This is an open-access article distributed under the terms of the Creative Commons Attribution License, which permits unrestricted use, distribution, and reproduction in any medium, provided the original author and source are credited.
Publication history:
SCI FORSCHEN JOURNALS
All Sci Forschen Journals are Open Access