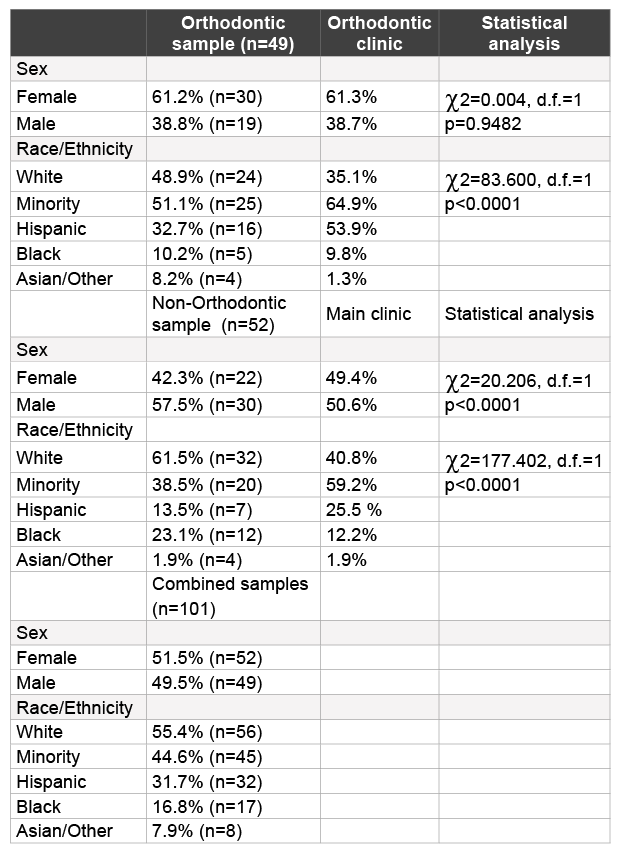
Table 1: Demographic analysis of study samples
Brandon J. Streiff1 Maryanne Seneviratne2 Karl Kingsley*3
1Department of Advanced Education Program in Orthodontics and Dentofacial Orthodpedics, Orthodontic Resident at the University of Nevada, Las Vegas - School of Dental Medicine, Las Vegas, Nevada, USA*Corresponding author: Dr. Karl Kingsley, Director of Student Research and Associate Professor at the University of Nevada, Las Vegas - School of Dental Medicine, Nevada, 89106, USA, Tel: (702) 774-2623; E-mail: Karl.Kingsley@unlv.edu
Orthodontic therapy in the United States has become routine among teenagers and increasing among adults. Despite these positive developments, orthodontic treatment has often been associated with changes to the oral environment, which may increase disease risk. Although numerous studies have demonstrated the causal link between Streptococcus mutans and carious lesions, more recent evidence suggest that it only constitutes part of a much larger oral microbial community. Several recent studies have demonstrated the presence of newly characterized cariogenic pathogen, the anaerobic Gram-positive bacillus Scardovia wiggsiae. This retrospective study of previously collected saliva samples originated with a convenience sample of pediatric and adult patients, previously recruited from the University of Nevada Las Vegas-School of Dental Medicine (UNLV-SDM) clinics. More than one hundred saliva samples from adult orthodontic (n=49) and non-orthodontic (n=52) patients were selected for inclusion in this study. All DNA extracted from these samples was subsequently screened using PCR, which revealed the presence of S. mutans (SM), P. gingivalis (PG), and S. wiggsiae (SW), which differed in prevalence among non-Orthodontic and Orthodontic patients. In non-orthodontic patients nearly all of the PG-positive and SW-positive samples were also SM-positive samples. However, among orthodontic patients, none of the SW-positive samples were either SM- or PG-positive. In addition, further analysis of demographic variables revealed decayed-missing-filled teeth (DMFT) score, periodontal pocket depth (PPD), age, gender, and BMI did not vary between groups, which suggest continued research in this area will be needed to elucidate these findings.
Scardovia wiggsiae; Caries; Orthodontics
Orthodontic therapy has become routine, with approximately 1% of all young adults (under 30 years of age) and nearly 20% of all teenagers undergoing some form of orthodontic treatment at any given time in the United States [1,2]. Despite these impressive advances in the prevalence of orthodontic treatment in the US, many disparities remain to improve access for the underserved, including minorities, underinsured and uninsured [3-5]. Many orthodontic and other dental specialty programs are now actively seeking low income, minority and underserved populations in an effort to improve not only access to oral healthcare, but to facilitate increased oral health awareness, education and other resources [6-8].
Despite these positive developments and the incremental steps towards improved access, orthodontic treatment has often been associated with changes to the oral mucosa, gingiva and microbial communities, which may increase disease risk [9,10]. More specifically, many studies have generated and evaluated the evidence regarding increased risk for caries lesions during orthodontic therapy, which may disproportionately affect these underserved, low income and minority populations [11-13]. In fact, this research group has facilitated several studies of oral health among minorities and under-served within the pediatric, adult and orthodontic populations over the past few years that clearly demonstrate these patients may be at increased risk for oral complications due to barriers to access, lower levels of health literacy, lowered access to preventive dental care, and increased burden of cariogenic, periodontal and other oral pathogens [6,7,14-16].
Although numerous studies have demonstrated the causal link between Streptococcus mutans and caries lesions, more recent evidence suggest cariogenic pathogens, such as S. mutans, constitute part of a much larger oral microbial community that may disproportionately influence caries risk when minor constituents become imbalanced – a process known as dysbiosis [17,18]. Many of these microbial species are well-known and have been extensively studied in the context of the cariogenic process, including Streptococcus, Lactobacillus, Veillonella and Actinomyces species [19]. However, several recent studies have demonstrated the presence of newly characterized cariogenic pathogen, the anaerobic Gram-positive bacillus Scardovia wiggsiae [20-22].
Despite the breadth of caries research, these more recent studies have demonstrated that these previously undetected (novel) cariogenic pathogens, such as S. wiggsiae, may be present in the oral cavity and may confer additional risks and might alter the current understanding of caries screening [23-25]. However, only a few preliminary studies have focused on this newly detected organism, and the cariogenic potential this organism may pose during orthodontic treatment when cariogenic risk may be comparatively higher [23,25]. Based upon the paucity of evidence, the goal of this study was to screen saliva from adult orthodontic patients to evaluate the prevalence of S. wiggsiae for comparison with a group of adult patients without orthodontic appliances. The data collected may be among the first to evaluate the presence of this organism within an orthodontic population at a public dental school that serves predominantly minority and underserved patients.
The protocol for this retrospective study of previously collected saliva samples titled “The Prevalence of Oral Microbes in Saliva from the University of Nevada Las Vegas (UNLV) School of Dental Medicine (SDM) pediatric and adult clinical population” (Protocol#1502-5068M) was reviewed and approved by the UNLV Office for the Protection of Research Subjects (OPRS) Institutional Review Board (IRB) on February 6, 2015.
This retrospective study of previously collected saliva samples originated with a convenience sample of pediatric and adult patients, previously recruited from the UNLV-SDM clinics. In brief, all participants had previously provided Informed Consent prior to collection of demographic information and saliva samples. Exclusion criteria included patients (or their appointed guardian) who declined to participate.
In the original study protocol, consented dental patients were given a sterile saliva 50 mL collection container for one sample. Samples were stored on ice until transfer to a biomedical laboratory for screening and analysis. Each of these samples was given a unique, randomly generated number to prevent research bias and any identifying information from being disclosed. The patient demographic and health information was also collected and given the matching randomly generated number for analytical purposes, but no patient-specific identifying information was subsequently available to any research team member.
For the purposes of this study, all previously collected saliva samples, which contained both shed epithelial cells and bacterial cells, were centrifuged for 10 minutes at 2,100 g (RCF) and the pellet washed with 1X phosphate-buffered saline (PBS) (Hy Clone: Logan, Utah, USA) and resuspended in 5 mL of 1X PBS. Epithelial cell number was determined using Trypan Blue (Fisher Scientific: Fair Lawn, New Jersey, USA) using a Zeiss Axiovert 40 inverted microscope (Carl Ziess, Inc: Thornwood, New York, USA) and a hemacytometer (Fisher Scientific: Fair Lawn, New Jersey, USA). To determine if any samples harbored the cariogenic pathogen of interest –S. wiggsiae or SW, DNA was isolated from the saliva sample using the Genomic Prep DNA isolation kit (Amersham Biosciences: Buckinghamshire, United Kingdom) and the procedure recommended by the manufacturer for blood and tissue, which recommends a minimum of 3.5 × 105 cells [14-16]. DNA was resuspended and stored in 50 µL DNA Hydration Solution (Amersham Biosciences: Buckinghamshire, United Kingdom) at 4C. DNA purity was calculated using ratio measurements of absorbance at 260 and 280 nm (A260/A280 ratio between 1.59 and 2.0).
DNA from each sample was then used to perform PCR with the Fisher exACTGene complete PCR kit (Fisher Scientific: Fair Lawn, New Jersey, USA) and a Mastercycler gradient thermocycler (Eppendorf: Hamburg, Germany) using the following primers for S. wiggsiae, S. mutans, P. gingivalis, 16S rRNA and glyceraldehyde- 3- phosphate dehydrogenase
(GAPDH) (SeqWright: Houston, Texas, USA) [14,16,20,21]:
GAPDH forward primer, ATCTTCCAGGAGCGAGATCC;
GAPDH reverse primer, ACCACTGACACGTTGGCAGT;
16S rRNA universal primer, ACGCGTCGACAGAGTTTGATCCTGGCT;
16S rRNA universal primer, GGGACTACCAGGGTATCTAAT;
S. mutans forward primer, GCCTACAGC TCAGAGATGCTATTCT;
S. mutans reverse primer, GCCATACACCACTCATGAATTGA;
P. gingivalis forward primer, TACCCATCGTCGCCTTGGT;
P. gingivalis reverse primer, CGGACTAAAACCGCATACACTTG;
S. wiggsiae forward primer, GTGGACTTTATGAATAAGC;
S. wiggsiae reverse primer, CTACCGTTAAGCAGTAAG;
DNA standards obtained from standardized control cells, human gingival fibroblasts (0.3-0.5 × 106 cells/mL), approximating the range of cell concentrations observed in the saliva samples were used to establish the minimum threshold (CT) and saturation (CS) cycles required for calibration and concentration comparisons using relative endpoint PCR (RE-PCR). GAPDH signal detection above background or CT required a minimum of ten cycles (C10), with saturation or CS observed at C50.
In addition, the oral bacterial cell lines Streptococcus mutans (S. mutans or SM) (NCTC-10449) and Porphyromonas gingivalis (P. gingivalis or PG) (FDC-381) were also obtained from ATCC (Manassas, VA). In brief, cells were thawed, streaked and cultured on their respective agar plates from Difco (Sparks, MD) according to the manufacturer protocol [14]. Colonies of each were then plated and grown overnight at 37C on Trypticase soy agar; SM plates were supplemented with 5% defibrinated sheep’s blood and PG plates were supplemented with 1% yeast extract. Single plate colonies were then selected and inoculated into liquid broth cultures; Trypticase soy broth for SM and supplemented tryptic soy broth for PG and then incubated overnight at 37C. Aliquots of bacterial suspensions were subsequently used to inoculate growth standards. Standard curves were created using spectrophotometric absorbance measurements of optical density (OD) at 650 nm and enumeration of colony forming units (CFU) [14,24].
Turbidity resulting in an OD of 0.8 corresponded to 5.0 × 107 CFU/ mL for both bacterial cell lines used. Serial dilutions were prepared for final concentrations of 5.0 × 106 , 105 , 104 and 103 CFU/mL to establish RE-PCR standards for SM and PG corresponding with the most current understanding of microbial saliva concentrations as biomarkers for disease (including caries) risk, which are: 106 CFU/mL = very high risk; 105 CFU/mL = high risk; 104 CFU/mL = moderate risk, and < 103 CFU/mL = normal or average risk [14,26,27]. SM and PG signal detection or CT above normal or average risk required a minimum of twenty five cycles (C25), with saturation or CS observed at C45. Based upon the CT data for GAPDH at C10 and for SM and PG at C25, RE-PCR was performed at C30, above the lower detection limit (CT), but below the observed saturation limits (C45-C50).
One µg of template DNA was then used for each reaction. The initial denaturation step ran for three minutes at 94°C. A total of 30 amplification cycles (C30) were run, consisting of 30 second of denaturation at 94°C, 60 seconds of annealing at 58°C, and 30 seconds of extension at 72°C. Final extension was run for five minutes at 72°C. The PCR reaction products were separated by gel electrophoresis using Reliant 4% NuSieve® 3:1 Plus Agarose gels (Lonza: Rockland, Maine, USA). Bands were visualized by UV illumination of ethidium-bromide-stained gels and captured using a Kodak Gel Logic 100 Imaging System and 1D Image Analysis Software (Eastman Kodak: Rochester, New York, USA).
To determine the appropriate sample size for this type of PCR screening for microbial composition using DNA extracted from saliva, the recovery rate from the sample-limited step of DNA extraction was used (90-95%) to establish the minimum expected difference of 0.10 or 10% [28] . Using a significance level of α= 0.05 and a power p = 0.80, a minimum sample size of fifty (N = 50) was calculated [29]. Descriptive statistics regarding the sample population were reported, and chi-square (χ2 ) analysis was performed to determine any significant differences in demographics between the sample group and the clinic population.
More than one hundred saliva samples from adult orthodontic (n=49) and non-orthodontic (n=52) patients were selected for inclusion in this study (Table 1). The demographic breakdown for all samples combined was nearly equally divided between males and females (51.5%, 49.5%), and between non-minority (white) and minorities (Hispanic, Black, Asian/ Other) (55.4%, 44.6%). More detailed analysis of the distribution from the orthodontic clinic revealed the sample to contain more females (61.2%) than males (38.8%), which closely resembled the overall distribution within the orthodontic clinic population (p=0.9482). An evaluation of the racial and ethnic demographics revealed nearly half of the samples were from non-minority patients (48.9%), which was significantly higher than in the overall orthodontic clinic population (35.1%) and was statistically significant (p<0.0001).
Table 1: Demographic analysis of study samples
The comparison or control group of adult non-orthodontic samples was comprised of fewer females (42.3%) than males (47.5%), which was different than their nearly equal distribution among the main clinic population, in general (p<0.0001). In addition, this analysis revealed the majority of samples were from non-minorities (61.5%), which was dissimilar from the main clinic population and also statistically significant (p<0.0001).
Each of the selected saliva samples were processed to isolate DNA for subsequent screening using PCR (Table 2). Using previously established protocols and methods, DNA was successfully isolated from all orthodontic and non-orthodontic saliva samples, yielding a recovery rate of 100% (n=101), which was within the acceptable range for DNA recovery according to the manufacturer (95-100%) and similar to results from previous studies [14-16,24]. DNA concentrations ranged from an average of 163.1 ng/uL from the orthodontic samples to 172.4 ng/uL from the non-orthodontic patient samples. DNA purity was calculated using ratio measurement of absorbance at 260 and 280 nm (A260/A280 ratio), which ranged between 1.59 and 2.0.The processing of all samples using PCR revealed the presence of both human (GAPDH) and bacterial (16S rRNA) DNA.
All DNA extracted from these samples was subsequently screened using PCR, which revealed the presence of S. mutans (SM), P. gingivalis (PG), and S. wiggsiae (SW), which differed in prevalence among non-Orthodontic and Orthodontic patients (Figure 1). For example, the proportion of patients with SM was significantly higher among Orthodontic patients (69%) than the control group (58%). Similar results were observed with PG, revealing a significantly larger percentage of Orthodontic patients with PG (55%) compared with non-Orthodontic patient samples (25%). However, the results of the SW screening revealed the prevalence was lower among the Orthodontic patient samples (14%) than the nonOrthodontic control group (19%). These differences between sample groups (Orthodontic, Non-Orthodontic) were found to be statistically significant (p<0.05).
To further analyze these results, each of the samples was cross referenced to determine if any samples were positive for one or more (multi-positive) of the pathogens evaluated (Figure 2). These results demonstrated that among the 58% of Non-Orthodontic (control) samples testing positive for SM, 21% were multi-positive for both SM and PG, while another 15% were SM and SW positive and a small fraction were SM-PG-SW positive (Figure 2A). Nearly all of the PG-positive and SW-positive samples were found among the SM-positive samples (Figure 2B), which suggests nearly two thirds (36% of 58%) demonstrated multi-positive results.
In contrast, a much higher proportion of the Orthodontic samples tested positive for SM (70%), although only a fraction (22%) were also found to be multi-positive (Figure 2C). In addition, the much higher percentage of PG-positive samples (55%) among the Orthodontic samples only partially overlapped with the SM-positive samples. Unexpectedly, none of the SW-positive samples were found to be SM- or PG-positive (Figure 2D).
Table 2: DNA isolation and recovery
Figure 1:
To determine the association and relationship with other demographic and health variables, data were sorted according to PCR screening results (Table 3). This analysis revealed that decayed-missing-filled teeth (DMFT) score did not vary significantly between the SM-, PG-, or SWpositive samples from the Non-Orthodontic and Orthodontic samples. In addition, no significant differences were found between periodontal pocket depth (PPD) when comparing the SM-, PG-, and SW-positive samples from these two groups. Further analysis, however, revealed that both DMFT score and PPD among the PG-positive samples were higher when compared with SM-, or multi-positive samples – regardless of whether the sample came from Orthodontic or Non-Orthodontic samples. Finally, the DMFT scores among the SW-positive samples were higher than those from SM-, PG- and multi-positive samples and PPDs greater than SM- or multi-positive samples. No other variables, including age, gender or BMI were differed significantly from the overall sample demographics.
The goal of this study was to screen saliva from adult orthodontic patients to evaluate the prevalence of SW for comparison with a group of adult patients without orthodontic appliances. The results of this study clearly demonstrate that differences in the prevalence of oral pathogens differed significantly among these two patient samples – revealing a comparatively higher prevalence of SM and PG in the Orthodontic sample. Interesting, SW prevalence was significantly lower in the Orthodontic sample – a finding which may be counterintuitive based upon the available information about this cariogenic organism [22,25].
Further analysis revealed that many of these samples in the NonOrthodontic group were multi-positive, with several differing combinations of pathogens detected. Moreover, the vast majority of samples that were PG- or SM-positive were also found to be SM-positive – with the only major, single-positive group harboring SM. In contrast to the findings among the Orthodontic sample, however, none of the SWpositive samples were found to harbor either SM- or PG. In addition, a much greater proportion of PG-positive samples did not harbor SM. When combined with the overall higher proportion of SM and PG within this group, these data may suggest a disruption in the overall oral ecosystem that may facilitate the overgrowth of specific types of oral microbes, with corresponding declines in the overall prevalence of competing organisms.
Finally, the data revealed that DMFT score and PPD were higher among the PG- and SW-positive samples compared with SM- and multi-positive samples. As previous data have demonstrated that DMFT score and PPD may often be indicators of poor oral health and may be higher among underserved and minority patients, the data collected from the study may serve to reinforce and strengthen the resolve for oral health providers to increase knowledge and awareness of these important issues and to lower barriers to dental access and care [5-8]. Although the data do not suggest an increase in SW prevalence within the orthodontic population, these may be among the first to reveal that orthodontic therapy may be sufficient to disrupt the oral ecology and ecosystem enough to create conditions that favor one major type of cariogenic organism, such as SM or SW – but not both. The data is especially valuable as it serve to evaluate the presence of SW within an orthodontic population at a public dental school that serves predominantly minority and underserved patients [6,7,14,15].
Figure 2:
Table 3: Association and relationship with other demographic and health variables
Most other studies of SW to date have involved either the identification or detection of this organism from children with Severe Early Childhood Caries (SECC) [20-22]. In addition, the most recent studies of SW involve detection of SW directly from caries lesions [23,30,31], while only one other study to date has included any patients with orthodontic appliances [25]. However, this study evaluated the presence of SM and SW and association with white spot lesions (WSL) - a known risk factor for caries development, but did not provide any data to analyze the presence of SM or SW from individual patients. The current study is the only study to date that provides any evidence to describe the oral microbial composition from individual SW-positive orthodontic patients, which has provided some evidence to suggest a novel, previously undescribed phenomenon that this type of disruption in the oral ecology may be sufficient to create conditions favoring SM or SW growth, but not both.
Although these findings may be among the first to screen for S. wiggsiae among Orthodontic and Non-Orthodontic patients, there are some limitations to consider for future studies of this nature. First, the use of an existing saliva repository restricts some of the conclusions that can be drawn from these observations based upon the retrospective study design. In addition, although the original collection protocol included both Orthodontic and Non-Orthodontic patients, these studies relied upon a convenience sample of willing participants that were not randomly selected and may therefore have implicit self-selection bias [12,14]. Furthermore, these patient samples were collected at a single time point, which suggests no temporal conclusions can be made regarding the observed oral microbial prevalence.
Despite these limitations based upon the study design, these findings are novel and may be clinically significant, which suggests the planning and implementation of prospective studies to evaluate any temporal changes within these populations. Data that can elucidate this phenomenon and describe any temporal changes over time are of the upmost importance. As the first known study of this type, a pilot study design using existing saliva samples was the best available option to provide an initial baseline screening within this patient population and provides crucial evidence tosuggest the need for continued research in this area.
Download Provisional PDF Here
Aritcle Type: Research Article
Citation: Streiff BJ, Seneviratne M, Kingsley K (2015) Screening and Prevalence of the Novel Cariogenic Pathogen Scardovia wiggsiae among Adult Orthodontic and Non-Orthodontic Patient Saliva Samples. Int J Dent Oral Health 1(6): doi http://dx.doi.org/10.16966/2378-7090.159
Copyright: © 2015 Streiff BJ, et al. This is an open-access article distributed under the terms of the Creative Commons Attribution License, which permits unrestricted use, distribution, and reproduction in any medium, provided the original author and source are credited.
Publication history:
All Sci Forschen Journals are Open Access