Figure 1: Schematic diagram of the Microbial Fuel Cell.
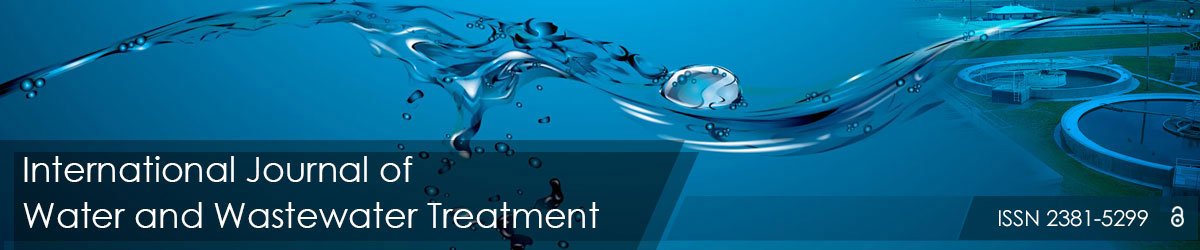
Full Text
JO Jeje* KT Oladepo SO Oyegoke
Department of Civil Engineering, Obafemi Awolowo University, Ile-Ife, Nigeria*Corresponding author: JO Jeje, Department of Civil Engineering, Obafemi Awolowo University, Ile-Ife, Nigeria, Tel: +234 (0) 8033 447 243; E-mail: jemails2000@yahoo.co.uk
This study undertook the generation of electricity from abattoir waste with the aim of eliminating the polluting effects of this waste. To achieve the aim of the study, three abattoirs were randomly selected within Ile-Ife in Osun State, southwest Nigeria. Using interactive and investigative methods, the average number of cows and goats that were slaughtered daily, weekly and monthly was established; and the amount of waste generated was subsequently estimated. Water samples were collected from water sources for the abattoirs. Wastewater samples were also collected at different carefully selected stages of the abattoir processes. The wastewater samples were exposed to air for 6 hours to 48 hours. The water and wastewater samples were then subjected to physico-chemical analysis, using standard procedures. Using standard method and appropriate materials, salt bridge was prepared and Microbial Fuel Cells (MFC) were assembled. Subsequently, electrical set up was done, abattoir wastewater introduced into the chamber and electricity generated and evaluated. Voltage, voltage drop, power and current readings were observed and recorded for a period of six (6) days (between 131-144 hours). Surveys revealed that an average of two (2) cows was slaughtered daily in each abattoir. Results showed that the quantity of waste generated from each abattoir per day stands at 100-120 kg per day. For fresh water samples, the pH fell within WHO standard (6.98 to 7.05); while the average pH value for the wastewaters showed that it is basic. Also, for fresh water samples, TS, TSS and BOD values met WHO standard (340 to 440 mg/l), 200 to 220 mg/l and 240 to 600 mg/l) respectively, while the values were excessively high for wastewaters. Generally, the voltage generated from waste with longest exposure to air had the least values. The study concluded that electricity generation is possible from abattoir waste using microbial fuel cells.
Abbatoir waste; Physico-chemical analysis; Salt bridge; Microbial Fuel Cells (MFC)
The continuous drive to increase meat production for the protein needs of the ever increasing world population has some pollution problems attached. Pollution arises from activities in meat production as a result of failure in adhering to Good Manufacturing Practices (GMP) and Good Hygiene Practices (GHP) [1]. Consideration is hardly given to safety practices during animal transport to the abattoir, during slaughter and during dressing [2]. Abattoir waste just like any other waste can be detrimental to humans and the environment if definite precautions are not taken. In Nigeria this waste are not properly managed. The abattoir or slaughter house dump the waste collected on a ground close to the slaughter house or wash it off to flow into a near-by stream or river resulting to excessive wastewater thereby causing water pollution. The waste dumped on the ground will attract flies and other insects as well as air pollution making the environment not conducive.
In Nigeria, managing waste disposal has become a major concern despite several attempts by successive governments and private organization in that direction [2]. For hygienic reasons abattoirs use large amount of water in processing operations; this produces large amount of wastewater. The major environmental problem associated with this abattoir waste is the large amount of suspended solids and liquid waste as well as odour generation [3]. Effluent from slaughterhouses has also been known to contaminate both surface and groundwater because during abattoir processing, blood, fat, manure, urine and meat tissue are lost to the wastewater streams [4,5].
Anthropogenic contaminations are evident: Effluents from various biological and chemical industries when untreated contribute to land, surface water and groundwater pollution. The world in the 21st century with the sustainable technology keeps improving in the management of resources, recirculation of waste in the generation of energy by causing little or no harm to the environment [6-8].
Materials and equipment
The materials used for this study were: water and waste water samples from identified abattoirs in Ile-Ife, southwest Nigeria; containers for water samples collection; chemicals and apparatus for physico-chemical analysis; anode electodes (100 mm × 100 mm aluminum mesh); anode chambers; and multimeter (Figure 1). Apart from the water samples, the materials were procured from the open market.
Methods
Identification and sampling of abattoir waste: Three abattoirs were randomly selected from Ile-Ife, southwest Nigeria. Their locations and descriptions are as follows: Oriloye Slaughterhouse (Eruobodo, Ibadan Road-Location 1. Olowo Slaughterhouse (Opposite Seventh Day Adventist Secondary School, Mayfair)-Location 2; and Alhaji Monyashau Slaughterhouse (Isale Agbara, Lagere-Modakeke Road)-Location 3. Interactive and investigative approach was used to ascertain the average number of cows and goats that were slaughtered daily, weekly and monthly in each of the abattoirs, and thus estimate the volume of waste generated. Using sterile containers, water samples were collected from the water sources of the abattoirs; wastewater samples were also carefully collected at each stage of the abattoir processes. Equal volumes of samples were collected from the identified locations; these samples were exposed to the atmospheric condition for certain number of hours to check the effect of the exposure on the bacteria. Sample collected at Location 1 was exposed for 6 hours; sample from Location 2 was exposed for 24 hours and sample from Location 3 was exposed for 48 hours. The samples were then taken to the Environmental Engineering Laboratory of the Department of Civil Engineering, Obafemi Awolowo University (OAU), Ile-Ife for physicochemical analysis.
Laboratory analysis
For the water and wastewater samples, the pH, temperature, BOD, TSS, and TS were determined in the Laboratory. The water samples were analyzed for chemical and bacteriological parameters using standard laboratory methods. Total Solids was analyzed using gravimetric method. The results of the analysis were then compared with World Health Organization [9].
Preparation of salt bridge
Salt bridge was made of 1000 ml 1 Molarity of potassium chloride (KCl) solution and 20 grams of Agar. The mixture was brought to boil at about 80°C and filled into the 100 mm long 50 mm diameter polyvinyl conduit before the temperature dropped below 40°C since it normally solidifies below that temperature [10-12]. The interior wall of the pipe was roughened sufficiently to enhance grip between the wall and the gel. This is because the salt bridge is only meant to allow the exchange of protons and not fluid contents of both the cathode and anode. The bridge linked the cathode and the anode chambers and undesired hole sealed up using epoxy.
Assembling of microbial fuel cells
Four two-chamber fuel cells were constructed (3 units of 4 liters and 1 unit of 2 liters). 100 mm × 100 mm aluminum mesh was used as the electrodes in all chambers. Copper wire of infinitesimal resistance was soldered to the aluminum mesh in order to establish the connection. With the wire itself, the mesh was suspended in the chamber at about 2 cm from the base of the chamber. The hole through which the wire was passed into the system was sealed using epoxy (Figure 1).
Setting up and generation of electricity
The system was set up as shown in Figure 1. Abattoir waste water was used to fill anode chambers up to three quarter of their volumes. The anode’s electrode was suspended vertically in the content at a distance of 5 cm from the surface while the cathode’s electrode was suspended (also vertically) in the freshwater/distilled water at a distance of 5 cm from the water surface. Water lost by evaporation may be replaced with double osmosis water. All MFCs were operated at room temperature (27°C). Potential (V) was measured at various times using a multimeter and recorded. Current (i) was calculated as:
i=V/R (1)
Where,
R is the external circuit resistor (RL. load resistor (1 k Ω) that could be easily replaced
Power (P) was calculated as:
P=iV (2)
The Power Density (PD) and current density (j) values were the P and i values normalized by the anode total geometric surface area. The maximum operating current density (jmax) was obtained from the curves of current density versus time.
Continuous voltage and current values were taken with the help of multi-meter and the procedures were repeated for six days (a total of 131 to 144 days).
Estimation and assessment of waste
The quantity of waste generated from the abattoirs was taken from the waste per cow (being the animal slaughtered mostly). On the average, each of the cows weighed 460 kg of which waste weighed 50-60 kg, including bones. On the average, two cows are killed daily. Therefore, the quantity of waste generated per these sizes of abattoirs per day stands at 100-120 kg per day. Out of this, approximately 270 kg of waste is being washed into the stream daily, based on the estimated 90 kg weight of bones and blood disposed on surrounding grounds excluding 360 kg of waste generated daily by the three abattoirs. However, according to Aniebo AO, et al. [3], the quantity of blood, intestinal content, waste tissue and bone that one cow and goat slaughtered can be generated for the three (3) abattoirs in lle-Ife is presented in table 1.
Abattoir Name | Cow/day | Blood/day (kg) | Intesti content/day (kg) | Bone/day (kg) | Waste tissue/day (kg) |
Ibadan road | 2 | 25.2 | 16 | 12.8 | 23.6 |
Seven day | 2 | 25.2 | 16 | 12.8 | 23.6 |
Lagere | 3 | 37.8 | 24 | 19.2 | 35.4 |
Total | 7 | 88.2 | 56 | 44.8 | 82.6 |
Table 1: Waste generated per day [3].
Results of water and wastewater analysis
The records of generated wastes is presented in table 1, while results of tests conducted on the water and wastewater samples are shown in table 2. The pH of the abattoirs waste and water samples were oscillating between slightly acidic and basic range with values ranging from 6.90- 7.30 for Location 1 abattoir; 6.90-7.30 for Location 2 abattoir and from 7.00-7.50 for Location 3 for both the waste and water samples (Table 2). However, the mean pH level of the abattoirs waste are slightly basic with values ranging between 7.20-7.50. The values of the pH for both the waste and water samples fall within the range of the pH standard of WHO (2006).
Location | Waste pH |
Water pH |
Waste Temp °C |
Water Temp °C |
Waste TS (mg/L) |
Water TS (mg/L) |
Waste TSS (mg/L) |
Water TSS (mg/L) |
Waste BOD5 (mg/L) |
Water BOD5 (mg/L) |
1 | 7.26 | 6.98 | 26.2 | 26.4 | 14740 | 440 | 7770 | 220 | 430 | 600 |
2 | 7.28 | 6.90 | 26.2 | 26.6 | 23.6 | 460 | 8180 | 270 | Nil | 580 |
3 | 7.43 | 7.05 | 26.1 | 26.4 | 11100 | 340 | 8690 | 200 | Nil | 240 |
W.H.O 2006 | 6.5-8.5 | 6.5-8.5 | 1000 | 1000 | 1000 | 1000 | 20 | 20 |
Table 2: Physico-chemical analysis of waste and water samples from the abattoirs.
Table 2 also shows that all the water samples collected from each of the abattoirs have low total solids values lower than WHO (2006) recommended value making the water safe in terms of total solid. The TS and TSS level of the abattoirs waste were found to be high, which is enough to cause pollution to the water body which it is being discharged to. Such high values of TS and TSS in the abattoir waste could be attributed to various solid by-products such as animal feces, soft tissue removed during slaughtering and cutting, fats, and soil from hides and hooves. Discharge of solids as well as blood and urine would increase the turbidity of water. Obtaining high BOD result in this study is expected since abattoir waste quality depends on the degree of blood, fats and other wastes.
Results of electricity generation
As shown in table 3, the voltage generated from Location 3 exposed for 48hours has the least voltage value which corresponds to previous work. Almost all of the rumen bacterial will die if they are exposed to oxygen-they are obligately anaerobic (Hungate, I975). From table 3, the voltage value for each location kept increasing from 0 hour of day 1 to 72 hours of day 3 and then a decrease in trend is observed to 144 hours of day 6. The voltage will continue to drop until a time where steady voltage is attained or a point of very small voltage as a result of complete decomposition of the nutrient in the abattoir waste by the bacterial. The maximum generated voltage at 72 hours was 299 mV and the minimum generated voltage of 100 mV was observed on 144 hours.
Location | 0 hrs (mV) |
11 hrs (mV) |
24 hrs (mV) |
35 hrs (mV) |
48 hrs (mV) |
59 hrs (mV) |
72 hrs (mV) |
83 hrs (mV) |
96 hrs (mV) |
107 hrs (mV) |
120 hrs (mV) |
131 hrs (mV) |
144 hrs (mV) |
1 | 160 | 164 | 195 | 209 | 263 | 268 | 299 | 287 | 231 | 219 | 171 | 147 | 100 |
2 | 206 | 198 | 189 | 162 | 145 | 135 | 70 | 74 | 80 | 77 | 74 | 72 | 68 |
3 | 104 | 97 | 83 | 64 | 12 | 9 | 25 | 22 | 25 | 22 | 23 | 22 | 11 |
Table 3: Voltage (mV) multimeter reading for 6 days in hours.
For Location 2 exposed for 24 hours as seen in table 3, the voltage generated at 0 hour of day 1 was higher than that of Location 1 exposed for 6 hours as a result of early decomposition of nutrient by the bacterial than that obtained in location 1. The voltage kept decreasing until 59 hours and a steady voltage between 65 mV-80 mV was observed from 72 hours of day 3 to 144 hours of day 6. This decrease when compared to the voltage value of location I follow the assumption made by Ichihashi O, et al. [12]. The maximum generated voltage at 0 hour was 206 mV and the minimum generated voltage of 68 mV was observed at 144 hours.
The sample collected in Location 3 was exposed for the longest time (48 hours), a voltage value of 104 mV was recorded at 0 hour sequel to exposition and gradually decreases until the 35th hour, then decreased at a faster rate to 12 mV at the 48th hour. There was an increase in the voltage value on the 72nd hour before obtaining a steady voltage value of 20-25 mv (Table 3). The voltage generated here is very small when compared to the other locations in accordance with Ichihashi O, et al. [12] assumption.
The small voltage generated according to Hungate (1975) implies that most of the bacterial are dead as a result of exposure to Oxygen. The maximum generated voltage at 0th hour was 104 mV and the minimum generated voltage of 9 mV was observed on 59th hour.
In lieu of the assumption stated in the proposal from the BOD experiment to invite various microorganisms responsible for decomposition of abattoir waste or perhaps, repopulation of the rumen bacterial. The results of the MFC carried out and from previous work have suggested the value obtained from the BOD experiment is not accurate due to faulty machine.
Potential drop was measured using the multimeter at 1000 Ω resistance at each time (hour) interval for the three locations exposed to the atmosphere at different duration. For further reading, resistance of 1000 Ω was kept constant and the values obtained were recorded (Table 4). Also, it was observed in location 1 sample exposed for 6 hours that the potential drop kept rising until the 72 hours where the maximum voltage was reached before decreasing to the 144 hours. Maximum voltage of 263 mV was obtained after at 72 hours of experiment while the minimum voltage of 85 mV was obtained at the 144 hours of the experiment. While in the case of location 2 sample, it was observed that the potential drop kept decreasing until the 72 hours of the experiment when the voltage values fluctuated between 55-65 mV. This can be linked to the longer exposure time than the sample in location 1. The higher value at the initial time is as a result of decomposed nutrient that had been converted to ions by the bacterial before dying gradually due to exposure to the atmosphere. Maximum voltage of 161 mV was obtained after at 0 hour of experiment while the minimum voltage of 56 mV was obtained at the 144 hours of the experiment.
Location | 0 hrs (mV) |
11 hrs (mV) |
24 hrs (mV) |
35 hrs (mV) |
48 hrs (mV) |
59 hrs (mV) |
72 hrs (mV) |
83 hrs (mV) |
96 hrs (mV) |
107 hrs (mV) |
120 hrs (mV) |
131 hrs (mV) |
144 hrs (mV) |
1 | 126 | 130 | 142 | 167 | 218 | 234 | 263 | 254 | 192 | 190 | 144 | 130 | 85 |
2 | 161 | 153 | 146 | 128 | 123 | 101 | 57 | 64 | 58 | 64 | 65 | 63 | 56 |
3 | 72 | 63 | 56 | 44 | 9 | 7 | 16 | 18 | 21 | 19 | 19 | 20 | 9 |
Table 4: Voltage drop of varied time of different location.
A decrease was observed in the location 3 sample up to 35 hours, then a sharp/rapid decrease at 48 hours and a sudden rise at 72 hours before maintaining a steady voltage. This irregularity is due to the exposure of the bacterial to the atmosphere since this bacterial are anaerobic and cannot survive in the presence of oxygen (Table 4). Maximum voltage of 72 mV was obtained after at 0 hours of experiment while the minimum voltage of 7 mV was obtained at the 59 hours of the experiment.
The resistance of the resistor was 1000 Ω. The value of current obtained from Ohms’ law is given in table 5. The value of the current cannot be obtained from the multimeter since the current generated was very small (micro Ampere, μA). Since Ohms has already established a relationship between current, voltage and resistance, Ohms’ law was used.
Location | 0 hrs (mA) |
11 hrs (mA) |
24 hrs (mA) |
35 hrs (mA) |
48 hrs (mA) |
59 hrs (mA) |
72 hrs (mA) |
83 hrs (mA) |
96 hrs (mA) |
107 hrs (mA) |
120 hrs (mA) |
131 hrs (mA) |
144 hrs (mA) |
1 | 0.126 | 0.130 | 0.142 | 0.167 | 0.218 | 0.234 | 0.263 | 0.254 | 0.192 | 0.190 | 0.144 | 0.130 | 0.085 |
2 | 0.161 | 0.153 | 0.146 | 0.128 | 0.123 | 0.101 | 0.057 | 0.064 | 0.058 | 0.064 | 0.065 | 0.063 | 0.056 |
3 | 0.072 | 0.063 | 0.056 | 0.044 | 0.009 | 0.007 | 0.016 | 0.018 | 0.021 | 0.019 | 0.019 | 0.020 | 0.009 |
Table 5: Current reading at varied exposure time for three locations.
From table 6, it can be seen that for the three locations, there is a constant increase up to 59 hours before decreasing to the 120 hours in the entire sample due to the fact that all the samples are exposed at equal time interval. The variation in the reading can be said to be as a result of disparity in the samples collected [13]. The maximum voltage was obtained at the as 59 hours and the minimum voltage was observed at 131 hours.
Location | 0-1 hrs (mV) |
11 hrs (mV) |
24 hrs (mV) |
35 hrs (mV) |
48 hrs (mV) |
59 hrs (mV) |
72 hrs (mV) |
83 hrs (mV) |
96 hrs (mV) |
107 hrs (mV) |
120 hrs (mV) |
131 hrs (mV) |
1 | 156 | 247 | 287 | 324 | 348 | 370 | 364 | 261 | 221 | 209 | 144 | 140 |
2 | 237 | 326 | 371 | 388 | 410 | 422 | 414 | 329 | 285 | 255 | 212 | 210 |
3 | 199 | 279 | 313 | 347 | 373 | 397 | 392 | 287 | 252 | 227 | 178 | 175 |
Table 6: Voltage reading of 4 litres sample of the three locations.
From the experiment result, it was observed the voltage for the bigger chamber is almost twice that of the smaller chamber. The voltage generated by the 2 litres chamber follows the same trend as that of the 4 litres (Table 6). The voltage generated in each of the six days is depicted in table 7. It is observed that there was a definitive increase in the generated voltage from 0 hour of day 1 to the 59 hour of day 3 and then a decline in trend is observed to 131 hours of day 6 before a steady voltage was observed. The maximum generated voltage at 59 hours was 231 mV and the minimum generated voltage of 59 mV was observed at 141 hours. The voltage measured was open circuit voltage since the external resistance is not used. Hence the voltage generated was due to internal impedance.
Time (hours) | 0-1 | 11 | 24 | 35 | 48 | 59 | 72 | 83 | 96 | 107 | 120 | 131 |
Voltage (mV) | 104 | 191 | 205 | 216 | 225 | 231 | 228 | 145 | 123 | 86 | 60 | 59 |
Table 7: Voltage reading of 2 litres sample.
The potential drop (Table 8) followed similar trend as the voltage. Maximum potential drop was obtained at the 59 hours and minimum potential drop at the 131 hours for both the 4 litres and 2 litres set up. Since the voltage drop has been obtained, current in mA can be obtained from Ohms’ law as in equation 1.
Location | 0 hrs (mV) |
11 hrs (mV) |
24 hrs (mV) |
35 hrs (mV) |
48 hrs (mV) |
59 hrs (mV) |
72 hrs (mV) |
83 hrs (mV) |
96 hrs (mV) |
107 hrs (mV) |
120 hrs (mV) |
131 hrs (mV) |
1 | 94 | 143 | 183 | 225 | 287 | 298 | 282 | 220 | 186 | 125 | 106 | 105 |
2 | 135 | 188 | 237 | 269 | 329 | 336 | 321 | 278 | 223 | 208 | 181 | 175 |
3 | 106 | 162 | 200 | 241 | 308 | 316 | 304 | 234 | 197 | 162 | 139 | 137 |
Table 8: Voltage drop reading of 4 litres evenly exposed sample of the three locations.
Table 9 shows that there was a definitive increase in the generated current from 0 hour to 59 hours and then a decline in trend is observed from 72 hours to 131 hours before obtaining stability in current. The maximum generated current at 59 hours was 0.336 mA and the minimum generated current of 0.135 mA was observed on 0 hour. The current measured was closed circuit voltage since the external resistance was used.
Location |
0 hrs (mA) |
11 hrs (mA) |
24 hrs (mA) |
35 hrs (mA) |
48 hrs (mA) |
59 hrs (mA) |
72 hrs (mA) |
83 hrs (mA) |
96 hrs (mA) |
107 hrs (mA) |
120 hrs (mA) |
131 hrs (mA) |
1 | 0.094 | 0.143 | 0.183 | 0.225 | 0.287 | 0.298 | 0.282 | 0.220 | 0.186 | 0.125 | 0.106 | 0.105 |
2 | 0.135 | 0.188 | 0.237 | 0.269 | 0.329 | 0.336 | 0.321 | 0.278 | 0.223 | 0.208 | 0.181 | 0.178 |
3 | 0.106 | 0.162 | 0.200 | 0.241 | 0.308 | 0.316 | 0.304 | 0.234 | 0.197 | 0.162 | 0.139 | 0.137 |
Table 9: Current reading of 4 litres sample of the three locations.
Rapid increase in voltage values was observed for the first 40 minutes from 22 mV to 110 mV and a drop of 1 mV between 40 minutes and 45 minutes was observed. From 45 minutes to 60 minutes a steady increase was observed in the voltage generated. Table 10 shows the Voltages of the 2 litres experiment in 1 hour.
Time (mins) | 1 | 10 | 15 | 20 | 25 | 30 | 35 | 40 | 45 | 50 | 55 | 60 |
Voltage (mV) | 22 | 46 | 62 | 71 | 82 | 91 | 104 | 110 | 109 | 115 | 117 | 122 |
Table 10: Voltage reading of 2 litres sample from 1 hour.
Also, table 11 showed that there was a definitive increase in the generated voltage from day 1 to day 3 and then a decline in trend is observed from day 4 to day 6. The maximum generated power at day 3 was 88.80 µW for Location 1, 112.90 µW for location 2, 99.86 µW for location 3 and the minimum generated power of 8.84 µW for Location 1, 18.23 µW for Location 2 and 11.24 µW for Location 3 were observed on day 1. The power measured was closed circuit power since the external resistance was used. Hence the power generated was not due to internal impedance, which seemed to be low in the range of watts (Table 11).
Location | 0 hrs (µW) | 11 hrs (µW) | 24 hrs (µW) | 35 hrs (µW) | 48 hrs (µW) | 59 hrs (µW) | 72 hrs (µW) | 83 hrs (µW) | 96 hrs (µW) | 107 hrs (µW) | 120 hrs (µW) | 131 hrs (µW) |
1 | 8.84 | 20.45 | 33.45 | 50.63 | 82.37 | 88.80 | 79.52 | 48.40 | 34.56 | 15.63 | 11.24 | 11.03 |
2 | 18.23 | 35.34 | 56.12 | 72.36 | 108.20 | 112.90 | 103.04 | 77.28 | 49.73 | 43.26 | 32.76 | 30.63 |
3 | 11.24 | 26.24 | 40.00 | 58.08 | 94.86 | 99.86 | 92.42 | 54.76 | 38.81 | 26.24 | 19.32 | 18.77 |
Table 11: Power generated by 4 litres for the three locations.
This study has established empirically that the major bacteria responsible for electrons scintillation (electrogenic) are those found in the intestines of the ruminant animals (specific reference to cow); and that these bacteria are not adversely affected by the fiery fur removal practice of the abattoirs selected, but are mostly adversely affected by aerobic condition. The study has proven that electricity generation is possible from abattoir waste using microbial fuel cells. Power can now be generated from what is considered as waste. Proportionality has also been established between the volume of microbial fuel cell and the possible voltage as shown in the result.
Electrodes in microbial fuel cell are best built as diaphragm covering almost the entire section of the chamber horizontally or vertically to enhance the power generation.
- Adeleye SA, Okorondu SI (2015) Bioelectricity from students’ hostel waste water using microbial fuel cell. Int J Biol Chem Sci 9: 103-1049. [Ref.]
- Alemma OA (2017) Disposal and Treatment of waste in Nigeria. In: Challenges managing waste disposal in Nigeria. The Guardian Magazine Lagos state.
- Aniebo AO, Wekhe SN, Okoli IC (2009) Abattoir blood waste generation in River state and its environmental implications in the Niger Delta. Toxicol Environ Chem 91: 619-625. [Ref.]
- Chen BY, Wang YM, Ng IS (2011) Understanding interactive characteristics of bioelectricity generation and reductive decolorization using Proteus hauseri. Bioresour Technol 102:1159- 1165. [Ref.]
- Choi JD, Chang HN, Han JI (2011) Performance of microbial fuel cell with volatile fatty acids from food wastes. Biotechnol Lett 33: 705- 714. [Ref.]
- Cucu A, Tiliakos A, Tanase L, Serban CE, Stamatin L, et al. (2016) Microbial fuel cell for nitrate reduction. Energy Procedia 85:156- 161. [Ref.]
- Fang C, Min B, Angelidaki I (2011) Nitrate as an oxidant in the cathode chamber of a microbial fuel cell for both power generation and nutrient removal purposes. Appl Biochem Biotechnol 164: 464- 474. [Ref.]
- Fang Z, Song HL, Cang N, Li XN (2015) Electricity production from Azo dye wastewater using a microbial fuel cell coupled constructed wetland operating under different operating conditions. Biosens Bioelectron 68: 135-141. [Ref.]
- WHO (2011) WHO Drinking Water Standard. [Ref.]
- Fernando E, Keshavarz T, Kyazze G (2012) Enhanced biodecolourisation of acid orange 7 by Shewanella oneidensis through co-metabolism in a microbial fuel cell. Int Biodeterior Biodegrad 72: 1-9. [Ref.]
- Gangadharan P, Nambi IM (2015) Hexavalent chromium reduction and energy recovery by using dual-chambered microbial fuel cell. Water Sci Technol 71: 353-358. [Ref.]
- Ichihashi O, Hirooka K (2012) Removal and recovery of phosphorus as struvite from swine wastewater using microbial fuel cell. Bioresour Technol 114: 303-307. [Ref.]
- Singh VP, Neelam S (2011) A survey report on Impact of Abattoir Activities Management on environments. Indian Journal of Veterinarians 6: 973-978.
Download Provisional PDF Here
Article Type: RESEARCH ARTICLE
Citation: Jeje JO, Oladepo KT, Oyegoke SO (2023) Generation of Electricity from Abattoir Waste Using Microbial Fuel Cell. Int J Water Wastewater Treat 9(1): dx.doi.org/10.16966/2381-5299.189
Copyright: © 2023 Jeje JO, et al. This is an open-access article distributed under the terms of the Creative Commons Attribution License, which permits unrestricted use, distribution, and reproduction in any medium, provided the original author and source are credited.
Publication history:
SCI FORSCHEN JOURNALS
All Sci Forschen Journals are Open Access