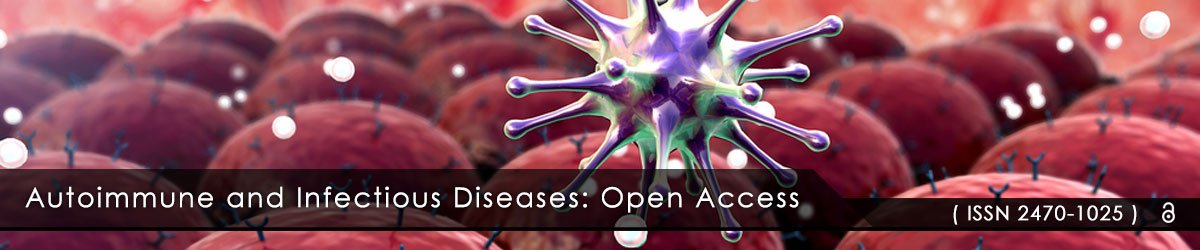
Full Text
Jianxun Song*
Department of Microbiology and Immunology, The Pennsylvania State University College of Medicine, Hershey, Pennsylvania, USA*Corresponding author: Jianxun Song, Department of Microbiology and Immunology, The Pennsylvania State University College of Medicine, Hershey, Pennsylvania, USA, E-mail: jus35@psu.edu
Pluripotent stem cells (PSCs) can be utilized to obtain a renewable source of healthy regulatory T cells (Tregs) to treat autoimmune arthritis as they have the ability to produce almost all cell types in the body, including Tregs. However, the right conditions for the development of antigen (Ag)- specific Tregs from PSCs (i.e., PSC-Tregs) remain unknown. An ongoing project will determine the mechanisms underlying the Ag-specific PSC-Treg treatments that aim to modulate tolerance in autoimmune arthritis. The knowledge gained from these studies will provide new insights into cellbased therapies in autoimmune arthritis, and advance the understanding of fundamental mechanisms underlying Treg differentiation.
Pluripotent stem cells; Autoimmune arthritis; Stem cells
Regulatory T cells (Tregs) are an integral component of the normal immune system and contribute to the maintenance of peripheral tolerance. Tregs can down-regulate immune responses and are essential for immune homeostasis. They can act as key effectors in preventing and treating rheumatoid arthritis (RA) [1,2].
Hematopoietic stem cell (HSC)-derived hematopoietic progenitors migrate into the thymus and develop into different types of T cells. The transcription factors Aire (largely expressed in thymic medullary epithelial cells - mTECs) and FoxP3 have key functions in clonal deletion and Treg selection [3]. There are links between Aire expression, FoxP3 upregulation and Treg selection; Aire deficiency affects the negative selection of self-reactive T cells, and FoxP3 controls the development and function of the naturally occurring Tregs(nTregs) [4]. Our laboratory has shown the development of stable Tregs from CD4+ T cells by over-expressing FoxP3 and bcl-xL [5].
Recent advances in the use of large-scale in vitro expansion of Tregs followed by in vivo re-infusion of these cells raises the possibility that this strategy may be successfully utilized for the treatment of rheumatoid arthritis (RA) [6]. Although polyclonally expanded populations of Tregs exhibit suppressive activity, antigen (Ag)-specific Tregs are more efficient at suppressing local autoimmune disorders such as RA, type-1 diabetes (T1D), inflammatory bowel diseases (IBD), allergic reactions and graftversus-host disease (GVHD) [7-11]. In addition, tissue/organ-associated Treg targeting stabilizes FoxP3 expression and avoids induction of a potentially detrimental systemic immunosuppression [12,13]. For Tregbased immunotherapy, in vitro generation of tissue/organ (e.g., synovium)- associated and non-terminally differentiated effector Tregs for in vivo reinfusion is an optimal approach. However, current methodologies are limited in terms of the capacity to generate, isolate, and expand a sufficient quantity of such Tregs from patients for therapeutic interventions.
A number of challenges exist in Treg-based immunotherapy:
Only low numbers of Tregs can be harvested from the peripheral blood mononuclear cells (PBMCs). CD4 and CD25 have been used to isolate Tregs for ex vivo expansion. CD4+CD25+ T cells are not homogenous and contain both Tregs and conventional effector T cells (Teffs). Current expansion protocols activate both Tregs and Teffs, and because it takes a longer time for Tregs to enter the S phase of cell cycle, Teffs outgrow Tregs [14]. In addition, Tregs can lose suppressive activity after repetitive stimulation with α-CD3 plus α--CD28 antibodies (Abs) with or without rIL-2 in vitro.
No approach to date has demonstrated the capacity to isolate the entire Treg population with 100% specificity from patients (the current clinical approach). Even FoxP3 or more recently Eos, a transcriptional factor that is considered the gold standard for identification of Tregs, is expressed transiently in some activated non-regulatory human T cells [15], highlighting the difficulty in both identifying and isolating a pure Treg population. The adoptive transfer of non-regulatory Teffs with Tregs has a potential to worsen autoimmune diseases.
Gene transduction of CD4+ T cells from PBMCs with Ag-specific T cell receptor (TCR) [16] or chimeric Ag receptor (CAR) [17] and/or TCR with FoxP3 elicits the generation of suppressive T cell populations [8] and overcomes the hurdle of the limited numbers of Ag-specific T cells. However, the engineered Tregs express endogenous and exogenous polyclonal TCRs, which reduce their therapeutic potential (the current experimental approach). Also, TCR mispairing is a concern with regards to the safety of TCR gene-transferred Tregs for clinical use, because the formation of new heterodimers of TCR can induce immunopathology [18]. Therefore, there is a need to improve this strategy and generate monoclonal Tregs.
The differentiation state of Tregs is inversely related to their capacity to proliferate and persist. The “right” Tregs resist terminal differentiation, maintain high replicative potential (e.g., expression of common- γ chain- γc , CD132), are less prone to apoptosis (e.g., low expression of PD-1), and have the ability to respond to homeostatic cytokines [19], which facilitates their survival. In addition, the “right” Tregs express high levels of molecules that facilitate their homing to lymph nodes (LNs), such as CD62L and CC-chemokine receptors (e.g., CCR4, CCR7), and maintain stability or plasticity under certain inflammatory conditions. Furthermore, after an effective immune response, the “right” Tregs persist and provide protective immunity.
Because there are too few cells, harvesting sufficient numbers of tissue-associated Tregs from PBMCs for TCR gene transduction can be problematic.
Taken together, strong arguments support the development of Tregbased therapies in autoimmune arthritis using engineered Tregs. While clinical trials show safety, feasibility, and potential therapeutic activity of Treg-based therapies using this approach, concerns about autoimmunity due to cross-reactivity with healthy tissues remains a major safety issue [20,21]. In addition, genetically modified Tregs using current approaches are usually intermediate or later effector Tregs [22], which only have shortterm persistence in vivo.
To date, pluripotent stem cells (PSCs) are the only source available to generate a high number of the “right” Tregs [23,24]. Human induced PSCs (iPSCs) can be easily generated from patients’ somatic cells by transduction of various transcription factors and exhibit characteristics identical to those of embryonic stem cells (ESCs) [25]. Many genetic methods as well as protein-based approaches have been developed to produce iPSCs with potentially reduced risks, including that of immunogenicity and tumorigenicity [26]. Because of the plasticity and the potential for an unlimited capacity for self-renewal, iPSCs have high potential for advancing the field of cell-based therapies.
Our laboratory was the first to show that the development of Agspecific iPSC-CTLs or iPSC-Tregs can be used for cell-based therapies of cancers and autoimmune disorders [23,24,27-30] other groups reported similar results [31-33]. We demonstrated that genetically modified iPSCs with Ag-specific TCR and the transcriptional factor FoxP3, followed by differentiation driven by Notch signaling can enable iPSCs to pass hematopoietic and T lineage differentiation checkpoints, resulting in the development of Ag-specific CD4+Tregs.We have developed a novel system to generate stable Ag-specific iPSC-Tregs. Our ongoing studies will validate this system and provide new insights into the methodologies and mechanistic requirements for efficient development of inflamed tissue-associated iPSC-Tregs. Once such strategies become available, there is potential to facilitate the generation of tolerance for autoimmune arthritis. Thus, important advances towards Treg-based immunotherapy in autoimmune arthritis are anticipated from the proposed studies.
PSCs are exposed to a number of signals responsible for their progression. Although the exact signals are not fully understood, part of the mechanism known to be critical for directing T-cell fate occurs via Notch signaling. The Notch is evolutionarily conserved; regulating cell fate decisions in a number of cell and tissue types. Ligand binding by members of the Jagged or Delta-like (DL) families results in the proteolytic cleavage and release of the intracellular fragment of the Notch heterodimer. Translocation to the nucleus then allows for its regulation of gene expression. Notch-1, specifically, is critical for the establishment of T-cell fate. The loss of function results in the blockade of T cell development and enhanced B cell production, while over-expression results in the blockade of B cell lymphopoiesis and leads to the generation of T cells [34]. However, the intracellular signaling pathways by which Notch signaling regulates the differentiation of Ag-specific PSC-Tregs remain unknown. PSCs co-cultured on a monolayer of the bone marrow (BM) stromal cell line OP9 cells transfected with the Notch ligand DL1 or 4 exhibits the ability to differentiate into most hematopoietic lineages and T cells [31]. Our studies will determine the critical regulations of Hes1 [35], Runx1 [36], and surviving [37] by Notch signaling during the development of autoAg-specific PSC-Tregs.
Although Ag-specific human iPSC-Tregs may have promising therapeutic effects in cell-based therapies, their efficiency is limited by the need to generate a large number of such cells using complex and expensive in vitro differentiation. In addition, the lengthy duration for generating human iPSCs may limit their use in individualized therapies. Alternatively, we will perform cell-based therapies using the TCR/FoxP3 gene-transduced iPSCs, which can differentiate into auto Ag-specific iPSC-Tregs in vivo and suppress autoimmune arthritis. We will perform arthritis induction before or after the adoptive transfer of the gene-transduced iPSCs. We will inject Notch agonists or recombinant cytokines (e.g., rIL-7, rFlt3L) to boost in vivo development of auto Ag-specific iPSC-Tregs.
In summary, a current roadblock to progress in the field is the lack of an efficient system to generate the “right” autoAg-specific Tregs that could be used for cell-based therapies in autoimmune arthritis. We propose the use of PSC-Tregs to address this limitation, allowing derivation of a large number of stable autoAg-specific PSC-Tregs for cell-based therapies. Development of such an approach provides an important step toward personalized therapies for autoimmune arthritis.
This project is funded, in part, under grants with the National Institute of Health Grant R01AI121180, R21AI109239 and K18CA151798, American Diabetes Association 1-16-IBS-281 and the Pennsylvania Department of Health using Tobacco Settlement Funds.
- Peres RS, Liew FY, Talbot J, Carregaro V, Oliveira RD, et al. (2015) Low expression of CD39 on regulatory T cells as a biomarker for resistance to methotrexate therapy in rheumatoid arthritis. Proc Natl Acad Sci U S A 112: 2509-2514. [Ref.]
- Chen M, Su W, Lin X, Guo Z, Wang J, et al. (2013) Adoptive transfer of human gingiva-derived mesenchymal stem cells ameliorates collagen-induced arthritis via suppression of Th1 and Th17 cells and enhancement of regulatory T cell differentiation. Arthritis Rheum 65: 1181-1193. [Ref.]
- Hossain DM, Panda AK, Manna A, Mohanty S, Bhattacharjee P, et al. (2013) FoxP3 acts as a cotranscription factor with STAT3 in tumorinduced regulatory T cells. Immunity 39: 1057-1069. [Ref.]
- Aschenbrenner K, D’Cruz LM, Vollmann EH, Hinterberger M, Emmerich J, et al. (2007) Selection of Foxp3+ regulatory T cells specific for self antigen expressed and presented by Aire+ medullary thymic epithelial cells. Nat Immunol 8: 351-358. [Ref.]
- Haque R, Lei F, Xiong X, Wu Y, Song J (2010) FoxP3 and Bcl-xL cooperatively promote regulatory T cell persistence and prevention of arthritis development. Arthritis Res Ther 12: R66. [Ref.]
- Hippen KL, Merkel SC, Schirm DK, Nelson C, Tennis NC, et al. (2011) Generation and large-scale expansion of human inducible regulatory T cells that suppress graft-versus-host disease. Am J Transplant 11: 1148-1157. [Ref.]
- van Herwijnen MJ, Wieten L, van der Zee R, van Kooten PJ, Wagenaar-Hilbers JP, et al. (2012) Regulatory T cells that recognize a ubiquitous stress-inducible self-antigen are long-lived suppressors of autoimmune arthritis. Proc Natl Acad Sci USA 109: 14134-14139. [Ref.]
- Wright GP, Notley CA, Xue SA, Bendle GM, Holler A, et al. (2009) Adoptive therapy with redirected primary regulatory T cells results in antigen-specific suppression of arthritis. Proc Natl Acad Sci U S A 106: 19078-19083. [Ref.]
- Sela U, Olds P, Park A, Schlesinger SJ, Steinman RM (2011) Dendritic cells induce antigen-specific regulatory T cells that prevent graft versus host disease and persist in mice. J Exp Med 208: 2489-2496. [Ref.]
- Bacher P, Kniemeyer O, Schonbrunn A, Sawitzki B, Assenmacher M, et al. (2013) Antigen-specific expansion of human regulatory T cells as a major tolerance mechanism against mucosal fungi. Mucosal Immunol 7: 916-928. [Ref.]
- Nguyen TL, Sullivan NL, Ebel M, Teague RM, DiPaolo RJ (2011) Antigen-specific TGF-beta-induced regulatory T cells secrete chemokines, regulate T cell trafficking, and suppress ongoing autoimmunity. J Immunol 187: 1745-1753. [Ref.]
- Van Belle TL, Ling E, Haase C, Bresson D, Urso B, et al. (2013) NKG2D blockade facilitates diabetes prevention by antigen-specific Tregs in a virus-induced model of diabetes. J Autoimmun 40: 66-73. [Ref.]
- Takiishi T, Korf H, Van Belle TL, Robert S, Grieco FA, et al. (2012) Reversal of autoimmune diabetes by restoration of antigen-specific tolerance using genetically modified Lactococcus lactis in mice. J Clin Invest 122: 1717-1725. [Ref.]
- Vogtenhuber C, O’Shaughnessy MJ, Vignali DA, Blazar BR (2008) Outgrowth of CD4low/negCD25+ T cells with suppressor function in CD4+CD25+ T cell cultures upon polyclonal stimulation ex vivo. J Immunol 181: 8767-8775. [Ref.]
- Sharma MD, Huang L, Choi JH, Lee EJ, Wilson JM, et al. (2013) An inherently bifunctional subset of Foxp3+ T helper cells is controlled by the transcription factor eos. Immunity 38: 998-1012. [Ref.]
- Perro M, Tsang J, Xue SA, Escors D, Cesco-Gaspere M, et al. (2010) Generation of multi-functional antigen-specific human T-cells by lentiviral TCR gene transfer. Gene Ther 17: 721-732. [Ref.]
- Porter DL, Levine BL, Kalos M, Bagg A, June CH (2011) Chimeric antigen receptor-modified T cells in chronic lymphoid leukemia. N Engl J Med 365: 725-733. [Ref.]
- Bendle GM, Linnemann C, Hooijkaas AI, Bies L, de Witte MA, et al. (2010) Lethal graft-versus-host disease in mouse models of T cell receptor gene therapy. Nat Med 16: 565-570. [Ref.]
- Gratz IK, Truong HA, Yang SH, Maurano MM, Lee K, et al. (2013) Cutting Edge: Memory Regulatory T Cells Require IL-7 and Not IL-2 for Their Maintenance in Peripheral Tissues. J Immunol 190: 4483-4487. [Ref.]
- Kuball J, Dossett ML, Wolfl M, Ho WY, Voss RH, et al. (2007) Facilitating matched pairing and expression of TCR chains introduced into human T cells. Blood 109: 2331-2338. [Ref.]
- van Loenen MM, de Boer R, Amir AL, Hagedoorn RS, Volbeda GL, et al. (2010) Mixed T cell receptor dimers harbor potentially harmful neoreactivity. Proc Natl Acad Sci U S A 107: 10972-10977. [Ref.]
- Kim YC, Zhang AH, Su Y, Rieder SA, Rossi RJ, et al. (2015) Engineered antigen-specific human regulatory T cells: immunosuppression of FVIII-specific T- and B-cell responses. Blood 125: 1107-1115. [Ref.]
- Haque R, Lei F, Xiong X, Bian Y, Zhao B, et al. (2012) Programming of regulatory T cells from pluripotent stem cells and prevention of autoimmunity. J Immunol 189: 1228-1236. [Ref.]
- Lei F, Haque R, Xiong X, Song J (2012) Directed differentiation of induced pluripotent stem cells towards T lymphocytes. J Vis Exp e3986. [Ref.]
- Kim JB, Sebastiano V, Wu G, Arauzo-Bravo MJ, Sasse P, et al. (2009) Oct4-induced pluripotency in adult neural stem cells. Cell 136: 411-419. [Ref.]
- Zhao T, Zhang ZN, Rong Z, Xu Y (2011) Immunogenicity of induced pluripotent stem cells. Nature 474: 212-215. [Ref.]
- Lei F, Haque R, Weiler L, Vrana KE, Song J (2009) T lineage differentiation from induced pluripotent stem cells. Cell Immunol 260: 1-5. [Ref.]
- Lei F, Zhao B, Haque R, Xiong X, Budgeon L, et al. (2011) In vivo programming of tumor antigen-specific T lymphocytes from pluripotent stem cells to promote cancer immunosurveillance. Cancer Res 71: 4742-4747. [Ref.]
- Haque M, Song J, Fino K, Sandhu P, Wang Y, et al. (2016) Melanoma Immunotherapy in Mice Using Genetically Engineered Pluripotent Stem Cells. Cell Transplant 25: 811-827. [Ref.]
- Haque M, Song J, Fino K, Sandhu P, Song X, et al. (2016) Stem cell-derived tissue-associated regulatory T cells ameliorate the development of autoimmunity. Sci Rep 6: 20588. [Ref.]
- Themeli M, Kloss CC, Ciriello G, Fedorov VD, Perna F, et al. (2013) Generation of tumor-targeted human T lymphocytes from induced pluripotent stem cells for cancer therapy. Nat Biotechnol 31: 928-933. [Ref.]
- Vizcardo R, Masuda K, Yamada D, Ikawa T, Shimizu K, et al. (2013) Regeneration of human tumor antigen-specific T cells from iPSCs derived from mature CD8(+) T cells. Cell Stem Cell 12: 31-36. [Ref.]
- Saito H, Okita K, Chang AE, Ito F (2106) Adoptive transfer of CD8+ T cells generated from induced pluripotent stem cells triggers regressions of large tumors along with immunological memory. Cancer Res 76: 3473-3483. [Ref.]
- Dervovic DD, Liang HC, Cannons JL, Elford AR, Mohtashami M, et al. (2013) Cellular and molecular requirements for the selection of in vitro-generated CD8 T cells reveal a role for notch. J Immunol 191: 1704-1715. [Ref.]
- Wendorff AA, Koch U, Wunderlich FT, Wirth S, Dubey C, et al. (2010) Hes1 is a critical but context-dependent mediator of canonical Notch signaling in lymphocyte development and transformation. Immunity 33: 671-684. [Ref.]
- Guo Y, Maillard I, Chakraborti S, Rothenberg EV, Speck NA (2008) Core binding factors are necessary for natural killer cell development and cooperate with Notch signaling during T-cell specification. Blood 112: 480-492. [Ref.]
- Lei F, Song J, Haque R, Xiong X, Fang D, et al. (2013) Transgenic expression of survivin compensates for OX40-deficiency in driving Th2 development and allergic inflammation. Eur J Immunol 43: 1914-1924. [Ref.]
Download Provisional PDF Here
Article Type: Mini Review
Citation: Jianxun Song (2016) Stem Cell-Derived Regulatory T Cells for Therapeutic Use in Arthritis. Autoimmun Infec Dis 2(3): doi http://dx.doi. org/10.16966/2470-1025.119
Copyright: © 2016 Song J. This is an open-access article distributed under the terms of the Creative Commons Attribution License, which permits unrestricted use, distribution, and reproduction in any medium, provided the original author and source are credited.
Publication history:
SCI FORSCHEN JOURNALS
All Sci Forschen Journals are Open Access